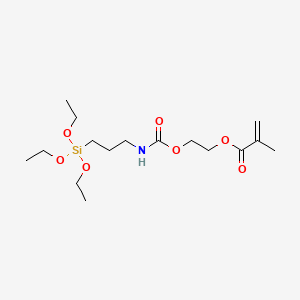
o-(Methacryloxyethyl)-n-(triethoxysilylpropyl)urethane
Overview
Description
o-(Methacryloxyethyl)-n-(triethoxysilylpropyl)urethane: is a multifunctional compound that combines the properties of methacrylate and alkoxysilane groups. This unique combination allows it to participate in both organic polymerization and inorganic sol-gel processes, making it highly versatile for various applications, particularly in the field of adhesives and coatings .
Mechanism of Action
Target of Action
o-(Methacryloxyethyl)-n-(triethoxysilylpropyl)urethane is a type of urethane methacrylate oligomer . It is primarily used in the production of crosslinked organic/inorganic hybrid nanovehicles . These nanovehicles are often used in drug delivery applications . Therefore, the primary targets of this compound are the organic/inorganic materials that it helps to crosslink.
Mode of Action
The compound acts as a crosslinking agent, helping to form stable structures for drug delivery . It interacts with its targets through a process known as triethoxysilyl-/trimethoxysilyl functionality . This process involves the formation of strong covalent bonds between the organic and inorganic materials, creating a stable, crosslinked structure .
Biochemical Pathways
The crosslinked structures it helps to form can be used to deliver drugs that do interact with biochemical pathways .
Pharmacokinetics
For example, the crosslinked structures can enhance the stability and control the release of the drug, affecting its absorption, distribution, metabolism, and excretion (ADME) properties .
Result of Action
The primary result of the compound’s action is the formation of stable, crosslinked organic/inorganic hybrid nanovehicles . These structures can enhance the stability of drug delivery systems, control the release of the drug, and potentially improve the drug’s efficacy .
Action Environment
The action of this compound can be influenced by environmental factors. For instance, the process of triethoxysilyl-/trimethoxysilyl functionality can be affected by the presence of water, as alkylsilanes react with hydroxyl groups . Additionally, the stability and efficacy of the crosslinked structures can be influenced by factors such as temperature and pH .
Biochemical Analysis
Biochemical Properties
The biochemical properties of o-(Methacryloxyethyl)-n-(triethoxysilylpropyl)urethane are largely determined by its urethane structure. The urethane reactions between a diisocyanate and an oligomeric polyol occur in the external phase, while the water-diisocyanate urea reactions occur at the oil–water interface . This compound interacts with various biomolecules, including enzymes and proteins, through these urethane bonds.
Cellular Effects
The cellular effects of this compound are primarily related to its role in the formation of polyurethane structures. Polyurethanes, including this compound, are known to present properties such as higher crystallinity, higher thermal stability, and increased fire resistance compared to polyether-based polyurethanes . They can also undergo hydrolysis when exposed to moisture and heat for a long time .
Molecular Mechanism
The molecular mechanism of action of this compound involves the formation of urethane bonds. The urethane reactions between a diisocyanate and an oligomeric polyol occur in the external phase, while the water-diisocyanate urea reactions occur at the oil–water interface . This leads to the formation of linear macromolecular structures that contribute to the unique properties of this compound .
Temporal Effects in Laboratory Settings
In laboratory settings, the effects of this compound can change over time. For instance, the polyurethane structures formed by this compound can undergo hydrolysis when exposed to moisture and heat for a long time . This can lead to changes in the properties of the compound and its effects on cellular function.
Preparation Methods
Synthetic Routes and Reaction Conditions: The synthesis of o-(Methacryloxyethyl)-n-(triethoxysilylpropyl)urethane typically involves the reaction of methacryloxyethyl isocyanate with triethoxysilylpropylamine. The reaction is carried out under anhydrous conditions to prevent hydrolysis of the alkoxysilane groups. The process can be summarized as follows:
Reactants: Methacryloxyethyl isocyanate and triethoxysilylpropylamine.
Conditions: Anhydrous environment, typically at room temperature.
Industrial Production Methods: Industrial production of this compound follows similar synthetic routes but on a larger scale. The process involves:
Bulk Reactors: Large-scale reactors to handle the reactants and maintain anhydrous conditions.
Purification: The product is purified through distillation or recrystallization to remove any unreacted starting materials and by-products.
Chemical Reactions Analysis
Types of Reactions:
Polymerization: The methacrylate group can undergo free-radical polymerization, forming cross-linked polymer networks.
Hydrolysis and Condensation: The alkoxysilane groups can hydrolyze in the presence of water, followed by condensation to form siloxane bonds, leading to the formation of an inorganic network.
Common Reagents and Conditions:
Polymerization: Initiators such as benzoyl peroxide or azobisisobutyronitrile (AIBN) are used under thermal or UV conditions.
Hydrolysis: Acidic or basic catalysts can be used to accelerate the hydrolysis and condensation reactions.
Major Products:
Polymer Networks: Cross-linked polymers with enhanced mechanical properties.
Siloxane Networks: Inorganic networks with improved thermal and chemical stability.
Scientific Research Applications
Coatings and Adhesives
O-(Methacryloxyethyl)-n-(triethoxysilylpropyl)urethane serves as a coupling agent in coatings and adhesives, enhancing adhesion to various substrates such as glass, metals, and ceramics. Its ability to improve the wetting properties of surfaces leads to better performance in protective coatings and bonding applications.
Application Area | Benefits |
---|---|
Coatings | Improved adhesion, enhanced durability |
Adhesives | Stronger bonds with inorganic substrates |
Biomaterials
This compound is utilized in the development of biocompatible materials, particularly in hydrogels for medical applications. It can be incorporated into hydrogel formulations to enhance mechanical properties and biocompatibility, making it suitable for use in tissue engineering and drug delivery systems.
- Case Study : A study demonstrated that incorporating this compound into hydrogel formulations improved the mechanical strength and swelling behavior of the hydrogels, making them more effective for tissue scaffolding applications .
Biomaterial Type | Application Examples |
---|---|
Hydrogels | Tissue scaffolds, drug delivery |
Surgical implants | Artificial organs, membranes |
Surface Modification
The compound is effective in modifying surfaces to achieve desired hydrophobic or hydrophilic characteristics. This property is particularly useful in creating anti-fogging surfaces or enhancing the performance of sensors.
- Case Study : Research indicates that treating glass surfaces with this compound significantly reduces water droplet adhesion, resulting in self-cleaning properties .
Surface Type | Modification Effect |
---|---|
Glass | Reduced surface tension |
Metals | Enhanced corrosion resistance |
Optical Applications
In the field of optics, this silane compound is used to enhance the performance of optical devices such as contact lenses. Its incorporation improves the clarity and comfort of lenses by providing better moisture retention and reducing protein deposition.
- Case Study : A patent outlines the use of this compound in manufacturing soft contact lenses that exhibit improved hydration properties and reduced irritation during wear .
Optical Product | Performance Improvement |
---|---|
Contact Lenses | Enhanced hydration |
Ophthalmic Devices | Reduced protein buildup |
Comparison with Similar Compounds
Methacryloxypropyltrimethoxysilane: Similar in structure but with different alkoxysilane groups.
Acryloxyethyltriethoxysilane: Contains an acryloxy group instead of a methacryloxy group.
Uniqueness:
Biological Activity
o-(Methacryloxyethyl)-n-(triethoxysilylpropyl)urethane (MTU) is a silane-based compound that has garnered attention in various fields, particularly in biomedical applications due to its unique chemical properties. This article focuses on the biological activity of MTU, examining its potential applications in drug delivery systems, tissue engineering, and other biomedical fields.
- Chemical Formula : C₁₆H₃₁NO₇Si
- Molecular Weight : 377.51 g/mol
- Density : 1.050 g/ml
- Boiling Point : 130°C
These properties contribute to MTU's functionality as a coupling agent and its reactivity in forming durable bonds with various substrates.
MTU functions primarily through its methacrylate and silane groups, which allow it to:
- Form covalent bonds with organic and inorganic materials.
- Enhance adhesion properties between different phases in composite materials.
- Participate in polymerization processes that can be utilized for creating hydrogels and other biomaterials.
Drug Delivery Systems
MTU has been explored for use in drug delivery due to its ability to form hydrogels that can encapsulate therapeutic agents. In a study, MTU-based hydrogels demonstrated controlled release characteristics, allowing for sustained drug delivery over time. The incorporation of MTU into hydrogel formulations has shown promise in improving the bioavailability of drugs while minimizing side effects .
Tissue Engineering
The application of MTU in tissue engineering is significant due to its biocompatibility and ability to form scaffolds that support cell growth. Research indicates that MTU can be used to create scaffolds that mimic the extracellular matrix, providing structural support for tissue regeneration. For instance, studies have shown that MTU-modified scaffolds enhance cell adhesion and proliferation, making them suitable for applications such as cartilage repair and wound healing .
Case Studies
- Hydrogel Formation : A study investigated the use of MTU in creating hydrogels for tissue engineering applications. The results indicated that hydrogels formed with MTU exhibited excellent mechanical properties and biocompatibility, supporting the growth of fibroblasts and promoting wound healing .
- Controlled Drug Release : In another case study, researchers developed a drug delivery system using MTU-based hydrogels loaded with anti-inflammatory drugs. The system demonstrated a sustained release profile over several days, effectively reducing inflammation in a model of rheumatoid arthritis .
- Scaffold Development : A recent investigation focused on MTU's role in developing biodegradable scaffolds for bone tissue engineering. The scaffolds showed enhanced mechanical strength and facilitated osteogenic differentiation of stem cells, indicating their potential for bone regeneration applications .
Comparative Analysis
Property | This compound | Other Silane Coupling Agents |
---|---|---|
Molecular Weight | 377.51 g/mol | Varies |
Biocompatibility | High | Moderate to High |
Drug Encapsulation Efficiency | High | Varies |
Mechanical Strength of Hydrogels | Excellent | Good |
Properties
IUPAC Name |
2-(3-triethoxysilylpropylcarbamoyloxy)ethyl 2-methylprop-2-enoate | |
---|---|---|
Source | PubChem | |
URL | https://pubchem.ncbi.nlm.nih.gov | |
Description | Data deposited in or computed by PubChem | |
InChI |
InChI=1S/C16H31NO7Si/c1-6-22-25(23-7-2,24-8-3)13-9-10-17-16(19)21-12-11-20-15(18)14(4)5/h4,6-13H2,1-3,5H3,(H,17,19) | |
Source | PubChem | |
URL | https://pubchem.ncbi.nlm.nih.gov | |
Description | Data deposited in or computed by PubChem | |
InChI Key |
HBFXZOMNDOKZCW-UHFFFAOYSA-N | |
Source | PubChem | |
URL | https://pubchem.ncbi.nlm.nih.gov | |
Description | Data deposited in or computed by PubChem | |
Canonical SMILES |
CCO[Si](CCCNC(=O)OCCOC(=O)C(=C)C)(OCC)OCC | |
Source | PubChem | |
URL | https://pubchem.ncbi.nlm.nih.gov | |
Description | Data deposited in or computed by PubChem | |
Molecular Formula |
C16H31NO7Si | |
Source | PubChem | |
URL | https://pubchem.ncbi.nlm.nih.gov | |
Description | Data deposited in or computed by PubChem | |
Molecular Weight |
377.50 g/mol | |
Source | PubChem | |
URL | https://pubchem.ncbi.nlm.nih.gov | |
Description | Data deposited in or computed by PubChem | |
CAS No. |
115396-93-5 | |
Record name | O-(Methacryloxyethyl)-N-(triethoxysilylpropyl)urethane | |
Source | European Chemicals Agency (ECHA) | |
URL | https://echa.europa.eu/information-on-chemicals | |
Description | The European Chemicals Agency (ECHA) is an agency of the European Union which is the driving force among regulatory authorities in implementing the EU's groundbreaking chemicals legislation for the benefit of human health and the environment as well as for innovation and competitiveness. | |
Explanation | Use of the information, documents and data from the ECHA website is subject to the terms and conditions of this Legal Notice, and subject to other binding limitations provided for under applicable law, the information, documents and data made available on the ECHA website may be reproduced, distributed and/or used, totally or in part, for non-commercial purposes provided that ECHA is acknowledged as the source: "Source: European Chemicals Agency, http://echa.europa.eu/". Such acknowledgement must be included in each copy of the material. ECHA permits and encourages organisations and individuals to create links to the ECHA website under the following cumulative conditions: Links can only be made to webpages that provide a link to the Legal Notice page. | |
Disclaimer and Information on In-Vitro Research Products
Please be aware that all articles and product information presented on BenchChem are intended solely for informational purposes. The products available for purchase on BenchChem are specifically designed for in-vitro studies, which are conducted outside of living organisms. In-vitro studies, derived from the Latin term "in glass," involve experiments performed in controlled laboratory settings using cells or tissues. It is important to note that these products are not categorized as medicines or drugs, and they have not received approval from the FDA for the prevention, treatment, or cure of any medical condition, ailment, or disease. We must emphasize that any form of bodily introduction of these products into humans or animals is strictly prohibited by law. It is essential to adhere to these guidelines to ensure compliance with legal and ethical standards in research and experimentation.