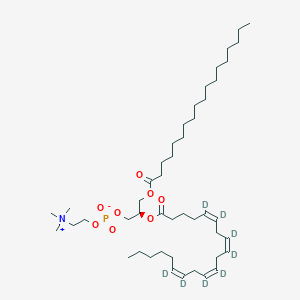
1-Stearoyl-2-Arachidonoyl PC-d8
Overview
Description
1-Stearoyl-2-Arachidonoyl-d8-sn-glycero-3-PC is a deuterium-labeled phospholipid. It is a derivative of 1-Stearoyl-2-Arachidonoyl-sn-glycero-3-PC, where hydrogen atoms are replaced with deuterium. This compound is used as an internal standard for the quantification of its non-deuterated counterpart in various analytical techniques such as gas chromatography-mass spectrometry (GC-MS) and liquid chromatography-mass spectrometry (LC-MS) .
Preparation Methods
The synthesis of 1-Stearoyl-2-Arachidonoyl-d8-sn-glycero-3-PC involves the incorporation of deuterium into the moleculeThe reaction conditions typically involve the use of deuterated reagents and solvents to ensure the incorporation of deuterium atoms .
Chemical Reactions Analysis
1-Stearoyl-2-Arachidonoyl-d8-sn-glycero-3-PC can undergo various chemical reactions, including:
Oxidation: This reaction can occur at the unsaturated bonds in the arachidonic acid moiety.
Reduction: The compound can be reduced to form different derivatives.
Substitution: The phosphatidylcholine group can be substituted with other functional groups under specific conditions.
Common reagents used in these reactions include oxidizing agents like hydrogen peroxide, reducing agents like sodium borohydride, and various catalysts for substitution reactions .
The major products formed from these reactions depend on the specific conditions and reagents used. For example, oxidation can lead to the formation of hydroperoxides, while reduction can yield saturated derivatives .
Scientific Research Applications
Metabolic Studies
SAPC-d8 is utilized in metabolic labeling experiments to trace lipid metabolism and dynamics within cells. The deuterium labeling allows for precise tracking using mass spectrometry techniques.
- Case Study : A study demonstrated the use of SAPC-d8 in tracing the synthesis and degradation pathways of phospholipids in cell cultures. The results indicated that SAPC-d8 could effectively label cellular membranes, allowing researchers to measure turnover rates of membrane lipids under various physiological conditions .
Lipid Signaling Research
The compound plays a crucial role in studying lipid signaling pathways, particularly those involving arachidonic acid derivatives, which are vital for inflammatory responses and cellular signaling.
- Case Study : Research focusing on diacylglycerol lipase activity used SAPC-d8 as a substrate to investigate the kinetics of lipid signaling molecules. The findings revealed that the incorporation of deuterated lipids improved the detection sensitivity of mass spectrometry methods for analyzing lipid metabolites .
Drug Development and Pharmacology
SAPC-d8 is increasingly being used in pharmacological studies to evaluate drug interactions with lipid membranes and their effects on cellular uptake mechanisms.
- Case Study : In a pharmacokinetic study, SAPC-d8 was employed to assess how certain drugs affect membrane fluidity and permeability. The study highlighted that drugs interacting with lipid membranes could alter the pharmacodynamics of therapeutic agents significantly .
Data Tables
Application Area | Description | Key Findings |
---|---|---|
Metabolic Studies | Tracing lipid metabolism | Effective labeling of cellular membranes |
Lipid Signaling | Investigating signaling pathways | Improved detection sensitivity in mass spectrometry |
Drug Development | Evaluating drug-membrane interactions | Significant alterations in drug pharmacodynamics |
Mechanism of Action
The mechanism of action of 1-Stearoyl-2-Arachidonoyl-d8-sn-glycero-3-PC involves its role as a phospholipid in cellular membranes. It can interact with various proteins and enzymes, influencing signaling pathways and cellular processes. The deuterium atoms in the molecule can affect its pharmacokinetic and metabolic profiles, making it a valuable tool in drug development .
Comparison with Similar Compounds
1-Stearoyl-2-Arachidonoyl-d8-sn-glycero-3-PC is unique due to its deuterium labeling, which distinguishes it from its non-deuterated counterpart, 1-Stearoyl-2-Arachidonoyl-sn-glycero-3-PC. Similar compounds include:
1-Stearoyl-2-Arachidonoyl-sn-glycero-3-PC: The non-deuterated version.
1-Stearoyl-2-Arachidonoyl-sn-glycerol: A diacylglycerol derivative.
1-Stearoyl-2-Arachidonoyl-phosphatidylcholine: Another phospholipid with similar fatty acid composition
Biological Activity
Overview
1-Stearoyl-2-Arachidonoyl PC-d8, a deuterium-labeled phospholipid, is a derivative of 1-Stearoyl-2-Arachidonoyl-sn-glycero-3-phosphocholine (PC). This compound is primarily utilized in scientific research as an internal standard for quantifying its non-deuterated counterpart in various analytical techniques, including gas chromatography-mass spectrometry (GC-MS) and liquid chromatography-mass spectrometry (LC-MS) . Its biological activity is significant due to its interaction with key enzymes and pathways involved in cellular signaling, inflammation, and metabolism.
Target Proteins
The primary targets of this compound include:
- Protein Kinase C (PKC) : This enzyme plays a crucial role in various cellular processes, including growth, differentiation, and apoptosis. The compound acts as an allosteric activator of PKC, enhancing its activity in response to diacylglycerol (DAG) levels .
- Cyclooxygenase (COX-2) : Involved in the synthesis of prostaglandins from arachidonic acid, COX-2 is associated with inflammation and pain responses. The activation of COX-2 by this compound contributes to the generation of pro-inflammatory mediators .
Biochemical Pathways
The activation of PKC and COX-2 leads to the modulation of several biochemical pathways:
- Cell Growth and Survival : By influencing PKC activity, the compound affects pathways related to cell proliferation and survival.
- Apoptosis : Changes in PKC signaling can lead to alterations in apoptotic processes, impacting cancer progression and treatment responses.
- Carcinogenesis and Metastasis : The compound's role in enhancing COX-2 activity suggests potential implications in cancer biology, particularly concerning tumorigenesis .
Cellular Effects
This compound influences various cellular functions through its effects on cell signaling pathways:
- Gene Expression : The compound modulates gene expression by activating transcription factors involved in inflammation and cell survival.
- Cellular Metabolism : It affects lipid metabolism and the generation of bioactive lipids, thereby influencing energy homeostasis within cells .
Case Studies
A study highlighted the specificity of arachidonoyl-containing substrates for certain enzymes. It demonstrated that mutations affecting the substrate binding site significantly reduced enzymatic activity against arachidonoyl substrates, emphasizing the importance of acyl chain specificity in lipid metabolism . This specificity is crucial for understanding how this compound interacts with metabolic pathways.
Comparative Analysis
The following table summarizes the biological activities of this compound compared to its non-deuterated counterpart:
Property | This compound | 1-Stearoyl-2-Arachidonoyl PC |
---|---|---|
Deuterium Labeling | Yes | No |
Primary Targets | PKC, COX-2 | PKC, COX-2 |
Role in Inflammation | Yes | Yes |
Use as Internal Standard | Yes | No |
Impact on Gene Expression | Significant | Significant |
Q & A
Basic Research Questions
Q. What is the role of 1-Stearoyl-2-Arachidonoyl PC-d8 in lipid quantification studies, and how does its deuterated structure enhance analytical accuracy?
- This deuterated phospholipid serves as an internal standard in mass spectrometry (MS)-based lipidomics, particularly for quantifying its non-deuterated counterpart, SAPC (1-Stearoyl-2-Arachidonoyl PC), in biological samples. The eight deuterium atoms at positions 5, 6, 8, 9, 11, 12, 14, and 15 provide a distinct mass shift, enabling precise differentiation from endogenous lipids during GC- or LC-MS analysis . Its use minimizes matrix effects and improves quantification accuracy by correcting for extraction efficiency and ionization variability .
Q. How should researchers validate the use of this compound as an internal standard in targeted lipidomics workflows?
- Validation requires assessing linearity, recovery, and precision:
- Linearity: Generate calibration curves using spiked samples across physiologically relevant concentration ranges (e.g., 0.1–100 ng/mL) to confirm proportional response .
- Recovery: Compare the measured concentration of spiked deuterated standard in biological matrices (e.g., plasma, cell lysates) against a solvent-only control to evaluate matrix interference .
- Precision: Perform intra- and inter-day replicates (n ≥ 5) to ensure coefficient of variation (CV) <15% .
Advanced Research Questions
Q. What are the key considerations for minimizing isotopic interference when using this compound in high-resolution mass spectrometry?
- Isotopic overlap can occur due to natural abundance of heavy isotopes (e.g., ¹³C, ¹⁵N) in endogenous lipids. To mitigate this:
- Use high-resolution MS (HRMS) with resolving power >30,000 to distinguish the deuterated standard’s exact mass from nearby peaks .
- Apply post-acquisition deisotoping algorithms or orthogonal techniques like ion mobility spectrometry to separate co-eluting isobars .
- Validate specificity by analyzing a "blank" matrix without the deuterated standard to confirm absence of endogenous interference .
Q. How can researchers resolve discrepancies in recovery rates of this compound across different lipid extraction protocols (e.g., Folch vs. Bligh-Dyer)?
- Recovery inconsistencies often stem from solvent polarity and phase partitioning differences:
- Folch (chloroform:methanol 2:1): Optimal for total lipid recovery but may retain hydrophilic contaminants, requiring additional washes with saline to improve purity .
- Bligh-Dyer (chloroform:methanol:water 1:2:0.8): Better for aqueous-rich samples but may under-extract long-chain phospholipids.
- Solution: Spike the deuterated standard post-extraction to correct for procedural losses or pre-spike to evaluate extraction efficiency directly. Compare both approaches in a pilot study to determine protocol suitability .
Q. What experimental design strategies are critical for studying oxidative derivatives of SAPC using this compound in inflammatory disease models?
- Key steps include:
- Model Selection: Use LDL oxidation assays or endothelial cell cultures exposed to pro-inflammatory cytokines (e.g., TNF-α) to simulate SAPC oxidation .
- Tracer Incorporation: Co-incubate deuterated SAPC with native LDL to track oxidation kinetics via LC-MS/MS, monitoring products like oxidized arachidonoyl moieties (e.g., hydroxyeicosatetraenoic acid-phosphatidylcholine) .
- Data Normalization: Express oxidized SAPC levels as ratios to the deuterated internal standard to account for batch-to-batch variability .
Q. Methodological Challenges and Contradictions
Q. How should researchers address conflicting reports on the stability of this compound in long-term storage?
- Stability variations may arise from storage conditions:
- Temperature: Store at −80°C under inert gas (argon) to prevent autoxidation. Avoid freeze-thaw cycles by aliquoting .
- Solvent: Dissolve in chloroform:methanol (1:1) with 0.01% BHT to inhibit radical-mediated degradation. Confirm stability via periodic LC-MS checks for degradation products (e.g., lyso-PC species) .
- Validation: Compare freshly prepared vs. stored standards to quantify degradation rates and establish acceptable storage durations .
Q. What statistical approaches are recommended for analyzing dose-response relationships in SAPC-mediated signaling studies using deuterated internal standards?
- Employ nonlinear regression models (e.g., sigmoidal dose-response curves) to estimate EC₅₀ values for SAPC-induced effects (e.g., monocyte adhesion). Use bootstrap resampling (n = 1000 iterations) to calculate 95% confidence intervals and assess model robustness . For small sample sizes, apply non-parametric tests (e.g., Mann-Whitney U) to compare groups, ensuring power analysis (α = 0.05, β = 0.2) is performed a priori .
Properties
IUPAC Name |
[(2R)-3-octadecanoyloxy-2-[(5Z,8Z,11Z,14Z)-5,6,8,9,11,12,14,15-octadeuterioicosa-5,8,11,14-tetraenoyl]oxypropyl] 2-(trimethylazaniumyl)ethyl phosphate | |
---|---|---|
Source | PubChem | |
URL | https://pubchem.ncbi.nlm.nih.gov | |
Description | Data deposited in or computed by PubChem | |
InChI |
InChI=1S/C46H84NO8P/c1-6-8-10-12-14-16-18-20-22-23-25-27-29-31-33-35-37-39-46(49)55-44(43-54-56(50,51)53-41-40-47(3,4)5)42-52-45(48)38-36-34-32-30-28-26-24-21-19-17-15-13-11-9-7-2/h14,16,20,22,25,27,31,33,44H,6-13,15,17-19,21,23-24,26,28-30,32,34-43H2,1-5H3/b16-14-,22-20-,27-25-,33-31-/t44-/m1/s1/i14D,16D,20D,22D,25D,27D,31D,33D | |
Source | PubChem | |
URL | https://pubchem.ncbi.nlm.nih.gov | |
Description | Data deposited in or computed by PubChem | |
InChI Key |
PSVRFUPOQYJOOZ-WHAFDBSCSA-N | |
Source | PubChem | |
URL | https://pubchem.ncbi.nlm.nih.gov | |
Description | Data deposited in or computed by PubChem | |
Canonical SMILES |
CCCCCCCCCCCCCCCCCC(=O)OCC(COP(=O)([O-])OCC[N+](C)(C)C)OC(=O)CCCC=CCC=CCC=CCC=CCCCCC | |
Source | PubChem | |
URL | https://pubchem.ncbi.nlm.nih.gov | |
Description | Data deposited in or computed by PubChem | |
Isomeric SMILES |
[2H]/C(=C(\[2H])/C/C(=C(/[2H])\C/C(=C(/[2H])\C/C(=C(/[2H])\CCCC(=O)O[C@H](COC(=O)CCCCCCCCCCCCCCCCC)COP(=O)([O-])OCC[N+](C)(C)C)/[2H])/[2H])/[2H])/CCCCC | |
Source | PubChem | |
URL | https://pubchem.ncbi.nlm.nih.gov | |
Description | Data deposited in or computed by PubChem | |
Molecular Formula |
C46H84NO8P | |
Source | PubChem | |
URL | https://pubchem.ncbi.nlm.nih.gov | |
Description | Data deposited in or computed by PubChem | |
Molecular Weight |
818.2 g/mol | |
Source | PubChem | |
URL | https://pubchem.ncbi.nlm.nih.gov | |
Description | Data deposited in or computed by PubChem | |
Retrosynthesis Analysis
AI-Powered Synthesis Planning: Our tool employs the Template_relevance Pistachio, Template_relevance Bkms_metabolic, Template_relevance Pistachio_ringbreaker, Template_relevance Reaxys, Template_relevance Reaxys_biocatalysis model, leveraging a vast database of chemical reactions to predict feasible synthetic routes.
One-Step Synthesis Focus: Specifically designed for one-step synthesis, it provides concise and direct routes for your target compounds, streamlining the synthesis process.
Accurate Predictions: Utilizing the extensive PISTACHIO, BKMS_METABOLIC, PISTACHIO_RINGBREAKER, REAXYS, REAXYS_BIOCATALYSIS database, our tool offers high-accuracy predictions, reflecting the latest in chemical research and data.
Strategy Settings
Precursor scoring | Relevance Heuristic |
---|---|
Min. plausibility | 0.01 |
Model | Template_relevance |
Template Set | Pistachio/Bkms_metabolic/Pistachio_ringbreaker/Reaxys/Reaxys_biocatalysis |
Top-N result to add to graph | 6 |
Feasible Synthetic Routes
Disclaimer and Information on In-Vitro Research Products
Please be aware that all articles and product information presented on BenchChem are intended solely for informational purposes. The products available for purchase on BenchChem are specifically designed for in-vitro studies, which are conducted outside of living organisms. In-vitro studies, derived from the Latin term "in glass," involve experiments performed in controlled laboratory settings using cells or tissues. It is important to note that these products are not categorized as medicines or drugs, and they have not received approval from the FDA for the prevention, treatment, or cure of any medical condition, ailment, or disease. We must emphasize that any form of bodily introduction of these products into humans or animals is strictly prohibited by law. It is essential to adhere to these guidelines to ensure compliance with legal and ethical standards in research and experimentation.