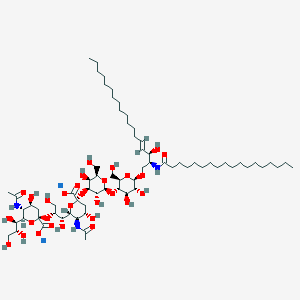
disialoganglioside GD3
- Click on QUICK INQUIRY to receive a quote from our team of experts.
- With the quality product at a COMPETITIVE price, you can focus more on your research.
Overview
Description
disialoganglioside GD3 is a glycosphingolipid composed of a ceramide lipid tail attached through glycosidic linkage to a glycan headgroup containing two sialic acid residues. This compound is highly abundant in the nervous system and plays a crucial role in cell signaling, modulation of membrane proteins, and ion channels. This compound is particularly significant in the brain, where it is involved in various physiological processes, including cell-cell communication and neuroprotection .
Mechanism of Action
Target of Action
Disialoganglioside GD3, also known as Ganglioside GD3 Sodium Salt, is a glycosphingolipid that is highly expressed in the nervous system . It is also found in neuroectoderm-derived tumors such as melanoma, neuroblastoma, and gliomas . The primary targets of this compound are cells that express significant levels of GD3 on their surface, such as human melanoma cell lines .
Mode of Action
this compound interacts with signaling molecules in lipid rafts . It has been shown to induce apoptosis in HuT-78 cutaneous T cell lymphoma cells in a concentration-dependent manner and disrupts the mitochondrial membrane potential . It also increases both cell proliferation and invasion in vitro when expressed in GD3-negative SK-MEL-28-N1 malignant melanoma cells .
Biochemical Pathways
this compound is synthesized by the addition of two sialic acid residues to lactosylceramide and can serve as a precursor to the formation of more complex gangliosides by the action of glycosyl- and sialyltransferases . It plays a role in the modulation of membrane proteins and ion channels, in cell signaling, and in the communication among cells . It is involved in tumor proliferation, invasion, metastasis, and immune responses .
Pharmacokinetics
It is known that this compound is a membrane-bound molecule, suggesting that its bioavailability would be primarily within the cell membrane environment where it is synthesized and functions .
Result of Action
The action of this compound results in various cellular effects. It induces apoptosis in certain cell types, disrupts the mitochondrial membrane potential, and increases cell proliferation and invasion in vitro . In addition, this compound-deficient adult mice exhibit progressive loss of the neural stem cell pool and impaired neurogenesis .
Action Environment
The action, efficacy, and stability of this compound can be influenced by various environmental factors. For instance, the expression of this compound can increase during development and in pathological conditions such as cancer . Furthermore, the presence of other molecules in the cell membrane environment can affect the function of this compound .
Biochemical Analysis
Biochemical Properties
Disialoganglioside GD3 plays a crucial role in various cellular processes, including cell adhesion, signal transduction, and immune modulation . It exerts its effects through interactions with cell surface receptors and intracellular signaling pathways . For instance, it has been reported that T cell activation via this compound is measured by the induction of tyrosine phosphorylation .
Cellular Effects
This compound has significant effects on various types of cells and cellular processes. It has been found to induce apoptosis in HuT-78 cutaneous T cell lymphoma cells in a concentration-dependent manner and disrupts the mitochondrial membrane potential when used at a concentration of 200 μM . Moreover, the expression of this compound in GD3-negative SK-MEL-28-N1 malignant melanoma cells increases both cell proliferation and invasion in vitro .
Molecular Mechanism
The molecular mechanism of action of this compound involves its interactions with biomolecules and changes in gene expression. For instance, it has been reported that this compound engagement resulted in phospholipase Cγ phosphorylation and calcium flux . When ras-associated events were examined, this compound signaling resulted in ras activation as determined by GDP/GTP conversion as well as dose-and time-dependent IP3 activation .
Temporal Effects in Laboratory Settings
It is known that this compound induces apoptosis in a concentration-dependent manner , suggesting that its effects may vary over time depending on the concentration used in the experiment.
Dosage Effects in Animal Models
The effects of this compound vary with different dosages in animal models. For instance, it has been reported that the intravenously injection of ganglioside GM1, a related compound, can treat the symptoms of Parkinson’s disease and acute ischemic stroke
Metabolic Pathways
This compound is involved in the metabolic pathways of glycosphingolipids. It is synthesized by the addition of two sialic acid residues to lactosylceramide . This process can serve as a precursor to the formation of more complex gangliosides by the action of glycosyl- and sialyltransferases .
Transport and Distribution
This compound is transported and distributed within cells and tissues. It is most abundant at the plasma membrane . Its hydrophobic ceramide tail inserts into the outer phospholipid leaflet, while the glycan headgroup extends outwardly and engages in cis (within the same membrane) as well as trans interactions (with molecules on other cells and in the extracellular space) that result in modulation of cell signaling and cell-to-cell communication .
Subcellular Localization
The subcellular localization of this compound is primarily on cell-surface microdomains . The drastic dose and composition changes during neural differentiation strongly suggest that this compound is not only important as biomarkers, but also are involved in modulating neural stem cell fate determination .
Preparation Methods
Synthetic Routes and Reaction Conditions: disialoganglioside GD3 is synthesized by the addition of two sialic acid residues to lactosylceramide. This process involves the action of glycosyl- and sialyltransferases, which facilitate the transfer of sialic acid residues to the glycan headgroup .
Industrial Production Methods: Industrial production of this compound typically involves extraction from bovine brain tissue, followed by purification processes to isolate the compound in its sodium salt form. The compound is then lyophilized to obtain a stable powder form for research and industrial applications .
Chemical Reactions Analysis
Types of Reactions: disialoganglioside GD3 undergoes various chemical reactions, including:
Oxidation: This reaction involves the addition of oxygen or the removal of hydrogen, leading to the formation of oxidized derivatives.
Reduction: This reaction involves the addition of hydrogen or the removal of oxygen, resulting in reduced forms of the compound.
Substitution: This reaction involves the replacement of one functional group with another, leading to the formation of substituted derivatives.
Common Reagents and Conditions:
Oxidation: Common reagents include hydrogen peroxide and potassium permanganate.
Reduction: Common reagents include sodium borohydride and lithium aluminum hydride.
Substitution: Common reagents include halogens and nucleophiles under various conditions.
Major Products Formed: The major products formed from these reactions include oxidized, reduced, and substituted derivatives of this compound, which can have different biological activities and applications .
Scientific Research Applications
disialoganglioside GD3 has a wide range of scientific research applications, including:
Chemistry: It is used as a precursor for the synthesis of more complex gangliosides and glycosphingolipids.
Biology: It plays a crucial role in cell signaling, differentiation, and apoptosis.
Medicine: this compound is studied for its potential therapeutic applications in neurodegenerative diseases, cancer, and immune modulation. .
Comparison with Similar Compounds
Ganglioside GM1: Another glycosphingolipid with a single sialic acid residue, known for its neuroprotective properties.
Ganglioside GD1a: Contains two sialic acid residues and is involved in cell signaling and neuroprotection.
Ganglioside GT1b: Contains three sialic acid residues and plays a role in cell-cell communication and neuroprotection
Uniqueness of disialoganglioside GD3: this compound is unique due to its specific structure and the presence of two sialic acid residues, which confer distinct biological activities. Its ability to induce apoptosis and regulate ion channels makes it a valuable compound for research and therapeutic applications .
Properties
IUPAC Name |
disodium;(2S,4S,5R,6R)-5-acetamido-6-[(1S,2R)-2-[(2S,4S,5R,6R)-5-acetamido-2-carboxylato-4-hydroxy-6-[(1R,2R)-1,2,3-trihydroxypropyl]oxan-2-yl]oxy-1,3-dihydroxypropyl]-2-[(2S,3R,4S,5S,6R)-2-[(2R,3S,4R,5R,6R)-4,5-dihydroxy-2-(hydroxymethyl)-6-[(E,2S,3R)-3-hydroxy-2-(octadecanoylamino)octadec-4-enoxy]oxan-3-yl]oxy-3,5-dihydroxy-6-(hydroxymethyl)oxan-4-yl]oxy-4-hydroxyoxane-2-carboxylate |
Source
|
---|---|---|
Source | PubChem | |
URL | https://pubchem.ncbi.nlm.nih.gov | |
Description | Data deposited in or computed by PubChem | |
InChI |
InChI=1S/C70H125N3O29.2Na/c1-5-7-9-11-13-15-17-19-20-22-24-26-28-30-32-34-52(84)73-44(45(80)33-31-29-27-25-23-21-18-16-14-12-10-8-6-2)41-95-65-59(89)58(88)61(51(40-77)97-65)98-66-60(90)64(56(86)49(38-75)96-66)102-70(68(93)94)36-47(82)54(72-43(4)79)63(101-70)57(87)50(39-76)99-69(67(91)92)35-46(81)53(71-42(3)78)62(100-69)55(85)48(83)37-74;;/h31,33,44-51,53-66,74-77,80-83,85-90H,5-30,32,34-41H2,1-4H3,(H,71,78)(H,72,79)(H,73,84)(H,91,92)(H,93,94);;/q;2*+1/p-2/b33-31+;;/t44-,45+,46-,47-,48+,49+,50+,51+,53+,54+,55+,56-,57+,58+,59+,60+,61+,62+,63+,64-,65+,66-,69+,70-;;/m0../s1 |
Source
|
Source | PubChem | |
URL | https://pubchem.ncbi.nlm.nih.gov | |
Description | Data deposited in or computed by PubChem | |
InChI Key |
LGRBZCLULGFXOD-DQMMGUQESA-L |
Source
|
Source | PubChem | |
URL | https://pubchem.ncbi.nlm.nih.gov | |
Description | Data deposited in or computed by PubChem | |
Canonical SMILES |
CCCCCCCCCCCCCCCCCC(=O)NC(COC1C(C(C(C(O1)CO)OC2C(C(C(C(O2)CO)O)OC3(CC(C(C(O3)C(C(CO)OC4(CC(C(C(O4)C(C(CO)O)O)NC(=O)C)O)C(=O)[O-])O)NC(=O)C)O)C(=O)[O-])O)O)O)C(C=CCCCCCCCCCCCCC)O.[Na+].[Na+] |
Source
|
Source | PubChem | |
URL | https://pubchem.ncbi.nlm.nih.gov | |
Description | Data deposited in or computed by PubChem | |
Isomeric SMILES |
CCCCCCCCCCCCCCCCCC(=O)N[C@@H](CO[C@H]1[C@@H]([C@H]([C@@H]([C@H](O1)CO)O[C@H]2[C@@H]([C@H]([C@H]([C@H](O2)CO)O)O[C@@]3(C[C@@H]([C@H]([C@@H](O3)[C@@H]([C@@H](CO)O[C@@]4(C[C@@H]([C@H]([C@@H](O4)[C@@H]([C@@H](CO)O)O)NC(=O)C)O)C(=O)[O-])O)NC(=O)C)O)C(=O)[O-])O)O)O)[C@@H](/C=C/CCCCCCCCCCCCC)O.[Na+].[Na+] |
Source
|
Source | PubChem | |
URL | https://pubchem.ncbi.nlm.nih.gov | |
Description | Data deposited in or computed by PubChem | |
Molecular Formula |
C70H123N3Na2O29 |
Source
|
Source | PubChem | |
URL | https://pubchem.ncbi.nlm.nih.gov | |
Description | Data deposited in or computed by PubChem | |
Molecular Weight |
1516.7 g/mol |
Source
|
Source | PubChem | |
URL | https://pubchem.ncbi.nlm.nih.gov | |
Description | Data deposited in or computed by PubChem | |
Disclaimer and Information on In-Vitro Research Products
Please be aware that all articles and product information presented on BenchChem are intended solely for informational purposes. The products available for purchase on BenchChem are specifically designed for in-vitro studies, which are conducted outside of living organisms. In-vitro studies, derived from the Latin term "in glass," involve experiments performed in controlled laboratory settings using cells or tissues. It is important to note that these products are not categorized as medicines or drugs, and they have not received approval from the FDA for the prevention, treatment, or cure of any medical condition, ailment, or disease. We must emphasize that any form of bodily introduction of these products into humans or animals is strictly prohibited by law. It is essential to adhere to these guidelines to ensure compliance with legal and ethical standards in research and experimentation.