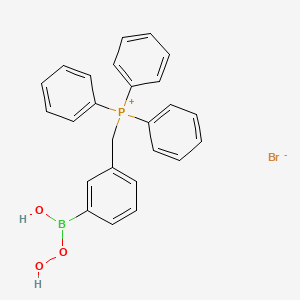
MitoB
Overview
Description
MitoB (3-boronobenzyl)triphenylphosphonium bromide is a mitochondria-targeted ratiometric probe designed to measure hydrogen peroxide (H₂O₂) levels in vivo . This conversion is approximately ten million times slower than endogenous H₂O₂ scavenging by mitochondrial peroxidases, ensuring minimal perturbation to physiological H₂O₂ levels . The MitoP/MitoB ratio, quantified via liquid chromatography-mass spectrometry (LC-MS/MS), reflects mitochondrial H₂O₂ concentrations over the exposure period . This compound has been validated in diverse models, including Drosophila, mice, and fish, making it a versatile tool for studying oxidative stress in ecological and biomedical contexts .
Preparation Methods
Synthetic Routes and Reaction Conditions
The synthesis of MitoB involves the conjugation of a triphenylphosphonium (TPP) cation to a 3-hydroxybenzyl moiety. The TPP group facilitates the accumulation of this compound within mitochondria due to its lipophilic nature and positive charge. The synthetic route typically includes the following steps:
Formation of the TPP Cation: The TPP cation is synthesized through the reaction of triphenylphosphine with an alkyl halide, such as methyl iodide, under reflux conditions.
Conjugation with 3-Hydroxybenzyl Bromide: The TPP cation is then reacted with 3-hydroxybenzyl bromide in the presence of a base, such as potassium carbonate, to form this compound.
Industrial Production Methods
Industrial production of this compound follows similar synthetic routes but on a larger scale. The process involves optimizing reaction conditions to ensure high yield and purity. Techniques such as recrystallization and chromatography are employed to purify the final product .
Chemical Reactions Analysis
Types of Reactions
MitoB primarily undergoes oxidation reactions within the mitochondrial matrix.
Common Reagents and Conditions
Oxidation: Hydrogen peroxide is the primary reagent that reacts with this compound. The reaction occurs within the mitochondrial matrix under physiological conditions.
Major Products
The major product formed from the oxidation of this compound is MitoP, which serves as an exomarker for hydrogen peroxide levels within mitochondria .
Scientific Research Applications
Ecological Studies
MitoB has been utilized to assess oxidative stress in ecological contexts. A notable study involved using this compound to measure H₂O₂ levels in brown trout (Salmo trutta). The findings indicated that H₂O₂ levels varied significantly between different tissues, enabling researchers to explore the role of ROS in life history trade-offs among aquatic organisms. The flexibility of the this compound method allows for varying exposure times and concentrations, making it suitable for diverse ecological research settings .
Mitochondrial Function Assessment
This compound has been instrumental in studying mitochondrial function and dynamics. By measuring H₂O₂ production within mitochondria, researchers can infer mitochondrial efficiency and health. For example, studies have shown that the accumulation of "old-mitochondria," which produce less ATP and more ROS, can be monitored using this compound . This application is crucial for understanding aging processes and age-related diseases.
Biomedical Research
In biomedical contexts, this compound is used to investigate the role of mitochondrial ROS in various diseases such as cancer, neurodegeneration, and cardiovascular conditions. The ability to measure H₂O₂ levels in real-time within living cells allows for a better understanding of disease mechanisms and potential therapeutic targets. For instance, research has demonstrated that this compound can help elucidate the signaling pathways affected by oxidative stress in cancer cells .
Case Studies
Study | Organism | Key Findings | Application |
---|---|---|---|
Cochemé et al. (2012) | Drosophila | Established baseline H₂O₂ levels in mitochondria | Model organism for oxidative stress research |
Research on Brown Trout (2017) | Salmo trutta | Variability of H₂O₂ levels across tissues; ecological implications | Ecological studies on oxidative stress |
Cardiovascular Disease Research (2022) | Various | Investigated mitochondrial dysfunction linked to ROS production | Potential therapeutic insights into heart disease |
Mechanism of Action
MitoB exerts its effects by accumulating within the mitochondrial matrix due to the lipophilic and positively charged TPP group. Once inside the mitochondria, the arylboronic moiety of this compound reacts with hydrogen peroxide to form MitoP. The ratio of MitoP to this compound is then measured to determine hydrogen peroxide levels. This mechanism allows for the precise quantification of mitochondrial hydrogen peroxide, providing insights into oxidative stress and mitochondrial function .
Comparison with Similar Compounds
MitoB vs. MitoPY1
MitoPY1 is a fluorescent probe that detects mitochondrial H₂O₂ via oxidative deboronation to MitoPY1ox, which emits strong fluorescence .
- Advantages of MitoPY1 :
- Limitations vs. This compound: Fluorescence intensity depends on probe concentration and environmental factors (e.g., pH, autofluorescence), reducing quantitative reliability . Limited utility in opaque tissues or whole organisms compared to this compound’s LC-MS/MS-based detection .
This compound vs. o-MitoPhB(OH)₂
o-MitoPhB(OH)₂, an isomer of this compound, reacts with both H₂O₂ and peroxynitrite (ONOO⁻), producing distinct products detectable via LC-MS/MS .
- Advantages of o-MitoPhB(OH)₂: Discriminates between H₂O₂ and ONOO⁻, useful in pathological conditions involving peroxynitrite .
- Limitations vs. This compound: Requires custom synthesis, whereas this compound is commercially available . Limited validation in ecological studies compared to this compound’s broad application across species .
This compound vs. DHE (Dihydroethidium)
Dihydroethidium (DHE) is a fluorescent probe for superoxide (O₂⁻), forming the fluorescent product 2-hydroxyethidium.
- Advantages of DHE :
- Limitations vs. This compound: Lacks mitochondrial specificity and cannot distinguish between ROS types . Fluorescence signals are confounded by non-specific oxidation and cellular heterogeneity .
This compound vs. Amplex Red
Amplex Red detects extracellular H₂O₂ via horseradish peroxidase (HRP)-catalyzed conversion to resorufin.
- Advantages of Amplex Red :
- Limitations vs. This compound: Cannot measure intracellular or mitochondrial H₂O₂ . Requires HRP, limiting its use in vivo .
Key Advantages of this compound
Mitochondrial Specificity : The TPP cation ensures >90% accumulation in mitochondria, enabling precise measurement of matrix H₂O₂ .
Ratiometric Quantification : The MitoP/MitoB ratio corrects for variations in probe uptake, extraction efficiency, and tissue heterogeneity .
Minimal Perturbation: Slow reaction kinetics avoid interference with endogenous H₂O₂ signaling or scavenging .
Longitudinal Measurement : this compound persists in tissues for up to 72 hours, enabling studies over days .
Limitations of this compound
Technical Complexity : Requires LC-MS/MS instrumentation and deuterated internal standards (d15this compound/d15MitoP) for quantification .
Cross-Reactivity : May react with peroxynitrite under pathological conditions, though this is rare in healthy tissues .
Tissue Heterogeneity : MitoP/MitoB ratios vary between tissues (e.g., liver vs. muscle) due to differences in mitochondrial density and ROS production .
Comparative Data Table
Research Findings
- Metabolic Rate : In brown trout, higher metabolic rates correlated with lower MitoP/MitoB ratios, suggesting efficient ROS scavenging in active individuals .
- Tissue-Specific ROS : White muscle showed 3.6-fold higher MitoP/MitoB ratios than liver in fish, reflecting tissue-specific mitochondrial activity .
Biological Activity
MitoB (Mitochondrial-targeted B) is a compound designed to selectively target mitochondria and measure reactive oxygen species (ROS), specifically hydrogen peroxide (), within living cells. It is a derivative of triphenylphosphonium (TPP) and serves as a redox-sensitive probe that can provide insights into mitochondrial function and oxidative stress in various biological contexts.
This compound operates by undergoing oxidation to form MitoP (Mitochondrial-targeted P), which allows for the quantification of levels based on the MitoP/MitoB ratio. This process is particularly important in assessing mitochondrial health and function, as elevated levels of ROS are often associated with cellular damage and various diseases.
Key Findings on this compound Activity
- Uptake and Localization : this compound rapidly accumulates in mitochondria, with studies showing a steady-state uptake of approximately 15 pmol per million Jurkat cells. The presence of FCCP, a mitochondrial uncoupler, reduced this uptake by about 40%, indicating that the majority of this compound is localized within the mitochondria rather than the cytosol .
- Oxidation and Measurement : The conversion of this compound to MitoP has been demonstrated in various models, including Drosophila and mice, where the MitoP/MitoB ratio increased in response to production during aerobic metabolism. This conversion was negligible in anaerobic conditions, confirming that this compound's oxidation is directly linked to mitochondrial activity .
- Ecological Applications : The this compound method has been adapted for ecological studies, allowing researchers to measure levels in living organisms over extended periods. For example, studies on brown trout have shown consistent levels across different tissues, demonstrating the method's versatility in various biological contexts .
Table 1: Summary of this compound Uptake and Conversion Studies
Study Model | Uptake Measurement | Conversion to MitoP | Notes |
---|---|---|---|
Jurkat Cells | 15 pmol/million cells | Yes | FCCP reduced uptake by ~40% |
Drosophila | Increased over 12 hours | Yes | Only converted under aerobic conditions |
Brown Trout | Consistent across tissues | Yes | Effective for ecological oxidative stress studies |
Table 2: Comparative Analysis of ROS Measurement Techniques
Technique | Sensitivity | Specificity | Duration |
---|---|---|---|
This compound Method | High | Specific to | Hours to days |
Traditional Fluorometry | Moderate | General ROS | Minutes |
Chemiluminescence | High | Specific to certain ROS | Seconds to minutes |
Case Study 1: Drosophila Model
In a study using Drosophila, researchers injected this compound and monitored the MitoP/MitoB ratio over time. The results indicated that aerobic metabolism significantly increased the ratio, correlating with production. In contrast, flies maintained in an anaerobic environment showed no change in this ratio, demonstrating the specificity of this compound for measuring mitochondrial ROS under physiological conditions .
Case Study 2: Brown Trout
The application of the this compound method was extended to brown trout, where it was used to assess oxidative stress related to metabolic rates. Fish were injected with this compound, and subsequent analysis revealed stable levels across different tissues over a 72-hour period. This study highlights the method's potential for ecological research and understanding oxidative stress in aquatic environments .
Q & A
Basic Research Questions
Q. What standardized protocols exist for applying the MitoB method to quantify mitochondrial H₂O₂ in vivo?
The this compound method involves administering a mitochondria-targeted probe (this compound) that reacts with H₂O₂ to form MitoP. Key steps include:
- Dosage optimization : Inject a standardized dose (e.g., 5 μM for cell cultures, 10 nmol/g for fish models) .
- Exposure duration : 24–72 hours for temperate ectotherms; shorter durations (e.g., 12 hours) for endotherms due to faster excretion rates .
- Validation : Use HPLC-MS to quantify MitoP/MitoB ratios. Ensure tissue-specific calibration (e.g., liver vs. pancreatic cells) .
- Contamination control : Verify no cross-contamination between injected and non-injected subjects in shared environments .
Q. How do researchers validate the specificity of this compound for H₂O₂ in complex biological systems?
Methodological validation includes:
- Negative controls : Use H₂O₂ scavengers (e.g., catalase) to confirm reduced MitoP formation .
- Competitive assays : Compare this compound reactivity with alternative ROS probes (e.g., MitoSOX Red) to rule out interference from superoxide or peroxynitrite .
- Tissue homogenization protocols : Centrifugation at 10,000×g to isolate mitochondrial fractions, minimizing cytoplasmic ROS interference .
Q. What are the critical parameters for reproducible this compound-based measurements across species?
- Temperature dependency : Adjust exposure times for ectotherms (e.g., 12°C for trout) vs. endotherms (e.g., 37°C for mammals) .
- Mass-normalized dosing : For field studies, use constant doses (not body mass-adjusted) to simplify logistics, but validate with post-hoc tissue concentration analyses .
- Time-normalized ratios : Calculate MitoP/(this compound + MitoP) over fixed intervals (e.g., 24 hours) to account for excretion rate variability .
Advanced Research Questions
Q. How can researchers resolve contradictions in MitoP/MitoB ratios when comparing in vitro vs. in vivo models?
- Experimental design : Conduct parallel in vitro (e.g., INS-1E cells) and in vivo (e.g., zebrafish) assays under matched H₂O₂ induction conditions (e.g., 25 mM glucose) .
- Data normalization : Use tissue-specific baseline ROS levels (e.g., pancreatic β-cells vs. hepatocytes) to contextualize ratios .
- Mechanistic follow-up : Pair this compound data with functional assays (e.g., mitochondrial membrane potential via TMRE) to distinguish technical artifacts from biological variability .
Q. What statistical approaches are recommended for analyzing time-course this compound data in longitudinal studies?
- Mixed-effects models : Account for individual variation in this compound excretion rates (random effects) while testing fixed effects like temperature or dose .
- Time-series normalization : Use area-under-the-curve (AUC) analysis for MitoP accumulation, adjusting for nonlinear excretion kinetics .
- Power analysis : For field studies, pre-determine sample sizes using pilot data on this compound detection limits (e.g., ≥5 fish per group to achieve 80% power) .
Q. How should researchers adjust this compound protocols for non-model organisms with unknown mitochondrial dynamics?
- Stepwise validation :
- Tissue pharmacokinetics : Measure this compound accumulation/excretion rates in pilot trials .
- Dose-response curves : Test 3–5 doses to identify the linear range of MitoP formation .
- Cross-species calibration : Compare this compound reactivity with phylogenetically related species with established protocols .
- Ethical considerations : Adhere to institutional guidelines for novel species, including minimal invasive sampling (e.g., fin clips for fish) .
Q. Data Contradiction & Validation
Q. How to address discrepancies between this compound-derived H₂O₂ levels and indirect oxidative stress markers (e.g., lipid peroxidation)?
- Triangulation : Combine this compound data with complementary assays (e.g., 8-OHdG for DNA damage, TBARS for lipid peroxidation) .
- Temporal resolution : Align measurement windows (e.g., acute H₂O₂ spikes vs. chronic oxidative damage) to reconcile differences .
- Mechanistic modeling : Use computational tools (e.g., Compartmental ROS Flux Models) to predict H₂O₂ contribution to downstream biomarkers .
Q. What steps ensure methodological transparency when publishing this compound studies?
- Supporting information : Provide raw HPLC-MS chromatograms, calibration curves, and tissue-specific limit-of-detection values .
- Replication guidelines : Detail injection techniques (e.g., intraperitoneal vs. intravenous), anesthesia protocols, and post-injection recovery times .
- Data availability : Share deidentified datasets via repositories like Dryad, adhering to FAIR principles (Findable, Accessible, Interoperable, Reusable) .
Properties
IUPAC Name |
[3-[hydroperoxy(hydroxy)boranyl]phenyl]methyl-triphenylphosphanium;bromide | |
---|---|---|
Source | PubChem | |
URL | https://pubchem.ncbi.nlm.nih.gov | |
Description | Data deposited in or computed by PubChem | |
InChI |
InChI=1S/C25H22BO3P.BrH/c27-26(29-28)22-12-10-11-21(19-22)20-30(23-13-4-1-5-14-23,24-15-6-2-7-16-24)25-17-8-3-9-18-25;/h1-19,27H,20H2;1H | |
Source | PubChem | |
URL | https://pubchem.ncbi.nlm.nih.gov | |
Description | Data deposited in or computed by PubChem | |
InChI Key |
MFIBJDFSRFHSFI-UHFFFAOYSA-N | |
Source | PubChem | |
URL | https://pubchem.ncbi.nlm.nih.gov | |
Description | Data deposited in or computed by PubChem | |
Canonical SMILES |
B(C1=CC(=CC=C1)C[P+](C2=CC=CC=C2)(C3=CC=CC=C3)C4=CC=CC=C4)(O)OO.[Br-] | |
Source | PubChem | |
URL | https://pubchem.ncbi.nlm.nih.gov | |
Description | Data deposited in or computed by PubChem | |
Molecular Formula |
C25H23BBrO3P | |
Source | PubChem | |
URL | https://pubchem.ncbi.nlm.nih.gov | |
Description | Data deposited in or computed by PubChem | |
Molecular Weight |
493.1 g/mol | |
Source | PubChem | |
URL | https://pubchem.ncbi.nlm.nih.gov | |
Description | Data deposited in or computed by PubChem | |
Disclaimer and Information on In-Vitro Research Products
Please be aware that all articles and product information presented on BenchChem are intended solely for informational purposes. The products available for purchase on BenchChem are specifically designed for in-vitro studies, which are conducted outside of living organisms. In-vitro studies, derived from the Latin term "in glass," involve experiments performed in controlled laboratory settings using cells or tissues. It is important to note that these products are not categorized as medicines or drugs, and they have not received approval from the FDA for the prevention, treatment, or cure of any medical condition, ailment, or disease. We must emphasize that any form of bodily introduction of these products into humans or animals is strictly prohibited by law. It is essential to adhere to these guidelines to ensure compliance with legal and ethical standards in research and experimentation.