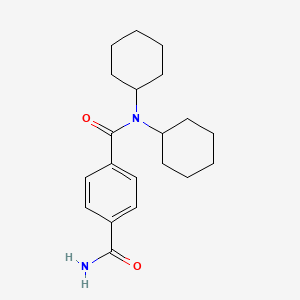
N,N-dicyclohexylterephthalamide
Description
N,N-dicyclohexylterephthalamide is a useful research compound. Its molecular formula is C20H28N2O2 and its molecular weight is 328.4 g/mol. The purity is usually 95%.
The exact mass of the compound this compound is 328.215078140 g/mol and the complexity rating of the compound is 413. The storage condition is unknown. Please store according to label instructions upon receipt of goods.
BenchChem offers high-quality this compound suitable for many research applications. Different packaging options are available to accommodate customers' requirements. Please inquire for more information about this compound including the price, delivery time, and more detailed information at info@benchchem.com.
Properties
IUPAC Name |
4-N,4-N-dicyclohexylbenzene-1,4-dicarboxamide | |
---|---|---|
Details | Computed by Lexichem TK 2.7.0 (PubChem release 2021.05.07) | |
Source | PubChem | |
URL | https://pubchem.ncbi.nlm.nih.gov | |
Description | Data deposited in or computed by PubChem | |
InChI |
InChI=1S/C20H28N2O2/c21-19(23)15-11-13-16(14-12-15)20(24)22(17-7-3-1-4-8-17)18-9-5-2-6-10-18/h11-14,17-18H,1-10H2,(H2,21,23) | |
Details | Computed by InChI 1.0.6 (PubChem release 2021.05.07) | |
Source | PubChem | |
URL | https://pubchem.ncbi.nlm.nih.gov | |
Description | Data deposited in or computed by PubChem | |
InChI Key |
OXJCOJXHCPVIPV-UHFFFAOYSA-N | |
Details | Computed by InChI 1.0.6 (PubChem release 2021.05.07) | |
Source | PubChem | |
URL | https://pubchem.ncbi.nlm.nih.gov | |
Description | Data deposited in or computed by PubChem | |
Canonical SMILES |
C1CCC(CC1)N(C2CCCCC2)C(=O)C3=CC=C(C=C3)C(=O)N | |
Details | Computed by OEChem 2.3.0 (PubChem release 2021.05.07) | |
Source | PubChem | |
URL | https://pubchem.ncbi.nlm.nih.gov | |
Description | Data deposited in or computed by PubChem | |
Molecular Formula |
C20H28N2O2 | |
Details | Computed by PubChem 2.1 (PubChem release 2021.05.07) | |
Source | PubChem | |
URL | https://pubchem.ncbi.nlm.nih.gov | |
Description | Data deposited in or computed by PubChem | |
DSSTOX Substance ID |
DTXSID90355197 | |
Record name | CBMicro_048206 | |
Source | EPA DSSTox | |
URL | https://comptox.epa.gov/dashboard/DTXSID90355197 | |
Description | DSSTox provides a high quality public chemistry resource for supporting improved predictive toxicology. | |
Molecular Weight |
328.4 g/mol | |
Details | Computed by PubChem 2.1 (PubChem release 2021.05.07) | |
Source | PubChem | |
URL | https://pubchem.ncbi.nlm.nih.gov | |
Description | Data deposited in or computed by PubChem | |
CAS No. |
15088-29-6 | |
Record name | CBMicro_048206 | |
Source | EPA DSSTox | |
URL | https://comptox.epa.gov/dashboard/DTXSID90355197 | |
Description | DSSTox provides a high quality public chemistry resource for supporting improved predictive toxicology. | |
Retrosynthesis Analysis
AI-Powered Synthesis Planning: Our tool employs the Template_relevance Pistachio, Template_relevance Bkms_metabolic, Template_relevance Pistachio_ringbreaker, Template_relevance Reaxys, Template_relevance Reaxys_biocatalysis model, leveraging a vast database of chemical reactions to predict feasible synthetic routes.
One-Step Synthesis Focus: Specifically designed for one-step synthesis, it provides concise and direct routes for your target compounds, streamlining the synthesis process.
Accurate Predictions: Utilizing the extensive PISTACHIO, BKMS_METABOLIC, PISTACHIO_RINGBREAKER, REAXYS, REAXYS_BIOCATALYSIS database, our tool offers high-accuracy predictions, reflecting the latest in chemical research and data.
Strategy Settings
Precursor scoring | Relevance Heuristic |
---|---|
Min. plausibility | 0.01 |
Model | Template_relevance |
Template Set | Pistachio/Bkms_metabolic/Pistachio_ringbreaker/Reaxys/Reaxys_biocatalysis |
Top-N result to add to graph | 6 |
Feasible Synthetic Routes
Q1: What is the primary function of N,N-dicyclohexylterephthalamide in the context of these research papers?
A1: this compound (DCTH) acts as a highly effective β-nucleating agent (β-NA) for isotactic polypropylene (iPP). [, , , , , , , ] This means it promotes the formation of the β-crystal form of iPP, which exhibits superior mechanical properties like impact strength compared to the more common α-form. [, ]
Q2: How does DCTH interact with iPP to induce β-crystal formation?
A2: DCTH provides a suitable surface for the epitaxial crystallization of β-iPP. This occurs through a two-dimensional match between specific crystallographic planes of DCTH and β-iPP. Specifically, the (110) plane of β-iPP aligns with the (001) plane of DCTH due to matching chain-axis and inter-chain directions. [] This epitaxial relationship makes the formation of β-iPP crystals more energetically favorable.
Q3: Does the size of the DCTH particles influence its nucleating efficiency?
A3: Yes, research suggests that the size of DCTH particles can influence its solubility in iPP and therefore affect the morphology of the resulting β-crystals. [] Different morphologies, such as flower-like agglomerates and β-transcrystalline entities, can form depending on the DCTH particle size, ultimately impacting the final mechanical properties of the iPP material. []
Q4: What are the practical implications of using DCTH in iPP?
A5: The addition of DCTH to iPP leads to enhanced toughness and impact strength in the final material. [, ] This makes DCTH-modified iPP highly desirable for applications requiring improved mechanical performance, such as hot-water pipes. [] The impact strength is directly related to the fraction of β-crystals (k) achieved. []
Q5: Does the presence of β-crystals induced by DCTH affect other properties of iPP?
A6: Research indicates that β-crystal formation in iPP, facilitated by DCTH, can significantly suppress space charge accumulation within the material. [] This effect is attributed to the unique morphology of β-crystals, which effectively weakens the mobility of charge carriers within the iPP matrix. []
Q6: Are there any limitations or challenges associated with using DCTH as a β-NA?
A7: One study highlights the reversible nucleation behavior of DCTH in polypropylene when combined with polyamide 12. [] This suggests that the effectiveness of DCTH as a β-NA can be influenced by the presence of other additives or specific processing conditions, potentially impacting its reliability in certain formulations.
Disclaimer and Information on In-Vitro Research Products
Please be aware that all articles and product information presented on BenchChem are intended solely for informational purposes. The products available for purchase on BenchChem are specifically designed for in-vitro studies, which are conducted outside of living organisms. In-vitro studies, derived from the Latin term "in glass," involve experiments performed in controlled laboratory settings using cells or tissues. It is important to note that these products are not categorized as medicines or drugs, and they have not received approval from the FDA for the prevention, treatment, or cure of any medical condition, ailment, or disease. We must emphasize that any form of bodily introduction of these products into humans or animals is strictly prohibited by law. It is essential to adhere to these guidelines to ensure compliance with legal and ethical standards in research and experimentation.