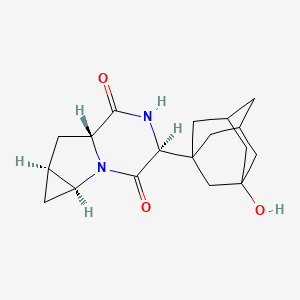
Saxagliptin Cyclic Amide
- Click on QUICK INQUIRY to receive a quote from our team of experts.
- With the quality product at a COMPETITIVE price, you can focus more on your research.
Overview
Description
Saxagliptin Cyclic Amide is a compound with the molecular formula C18H24N2O3 . It is also known by other names such as (2S,4S,6R,9S)-9-(3-Hydroxy-1-adamantyl)-1,8-diazatricyclo[4.4.0.02,4]decane-7,10-dione . It is used to improve the glycaemic control in type 2 diabetic patients .
Synthesis Analysis
The synthesis of Saxagliptin Cyclic Amide involves several steps. It starts from 1-adamantanecarboxylic acid, which is treated with sulfuric acid/nitric acid to get 3-hydroxy-1-adamantanecarboxylic acid. This is then treated with the one-pot method through acylation, condensation, and decarboxylation to obtain 3-hydroxy-1-acetyladamantane. Finally, it is oxidized by potassium permanganate (KMnO4) to get the target compound .Molecular Structure Analysis
The molecular structure of Saxagliptin Cyclic Amide includes a complex arrangement of carbon, hydrogen, nitrogen, and oxygen atoms. The exact mass of the compound is 316.17869263 g/mol .Chemical Reactions Analysis
Saxagliptin Cyclic Amide is a dipeptidyl peptidase-4 (DPP-4) inhibitor. It works by preventing the degradation of the incretin hormones, namely glucagon-like peptide-1 and glucose-dependent insulinotropic polypeptide. This increases glucagon-like peptide-1 levels, stimulates insulin secretion, and reduces the postprandial glucagon and glucose levels in the body .Physical And Chemical Properties Analysis
Saxagliptin Cyclic Amide has a molecular weight of 316.4 g/mol. It has 2 hydrogen bond donors and 3 hydrogen bond acceptors. Its rotatable bond count is 1. The topological polar surface area is 69.6 Ų .Scientific Research Applications
Evaluation of Drug-Induced Liver Injury Potential
Saxagliptin has been used in studies to evaluate its potential to cause drug-induced liver injury . In these studies, saxagliptin was found to covalently bind to the thiol groups of cysteine residues of endogenous proteins in vivo . This indicates the potential for saxagliptin to cause drug-induced liver injury .
Reactive Metabolite Identification
Research has been conducted to identify the reactive metabolites of saxagliptin . In these studies, saxagliptin and vildagliptin, which both contain a cyanopyrrolidine group, quickly reacted with L-cysteine to enzyme-independently produce thiazolinic acid metabolites . This saxagliptin–cysteine adduct was also found in saxagliptin-administered male Sprague–Dawley rats .
DPP-IV Inhibition
Saxagliptin is a highly potent, orally available reversible dipeptidyl peptidase IV (DPP-IV) inhibitor . It has been used in research to understand the mechanism of DPP-IV inhibition and its effects on glucose regulation .
Treatment of Type 2 Diabetes Mellitus
Saxagliptin has been approved by the FDA for the treatment of type 2 diabetes mellitus . It works by increasing the endogenous concentration of GLP-1, a hormone responsible for the glucose-dependent stimulation of insulin in the human body .
Synthesis of Free Base Monohydrate
The synthesis of the desired free base monohydrate of saxagliptin was challenging due to the thermodynamically favorable conversion of the free amine to the six-membered cyclic amidine . Research has been conducted to overcome this challenge and enhance the process robustness .
Kinetic and Mechanistic Insight
Research has been conducted to gain kinetic and mechanistic insight into the thermodynamically favored cyclization of saxagliptin to form the corresponding cyclic amidine . This research was crucial in developing a commercial synthesis that afforded saxagliptin with only trace levels of this key byproduct .
Safety and Hazards
Saxagliptin Cyclic Amide should be handled in a laboratory hood whenever possible. It should not be released into the environment. In case of eye contact, rinse immediately with plenty of water for at least 15 minutes and get medical attention. If ingested, clean mouth with water and drink afterwards plenty of water .
Mechanism of Action
Target of Action
Saxagliptin Cyclic Amide primarily targets the Dipeptidyl Peptidase-4 (DPP-4) enzyme . DPP-4 is responsible for degrading the intestinally derived hormones glucagon-like peptide (GLP)-1 and glucose-dependent insulinotropic polypeptide (GIP) . These hormones play a crucial role in regulating blood glucose levels.
Mode of Action
Saxagliptin forms a reversible, histidine-assisted covalent bond between its nitrile group and the S630 hydroxyl oxygen on DPP-4 . By inhibiting DPP-4, saxagliptin increases intact plasma GLP-1 and GIP concentrations, augmenting glucose-dependent insulin secretion .
Biochemical Pathways
The inhibition of DPP-4 by saxagliptin leads to an increase in the concentration of GLP-1 and GIP, which in turn enhances glucose-dependent insulin secretion . This results in better regulation of blood glucose levels. Saxagliptin can also covalently bind to the thiol groups of cysteine residues of endogenous proteins in vivo , indicating the potential for saxagliptin to cause drug-induced liver injury.
Pharmacokinetics
Saxagliptin is orally absorbed and can be administered with or without food . It is primarily metabolized by cytochrome P450 (CYP) 3A4/5 to an active metabolite, 5-hydroxy saxagliptin . The half-life of plasma DPP-4 inhibition with saxagliptin 5 mg is approximately 27 hours, supporting a once-daily dosing regimen . Saxagliptin is eliminated by a combination of renal and hepatic clearance .
Result of Action
The primary result of saxagliptin’s action is the improved regulation of blood glucose levels. This is achieved through the augmentation of glucose-dependent insulin secretion, facilitated by increased concentrations of GLP-1 and GIP .
Action Environment
The action of saxagliptin can be influenced by environmental factors. For instance, the formation of acidic polyethylene glycol (PEG) degradation products can lower the micro-environmental pH, which in turn lowers the formation of the main saxagliptin degradation product, epi-cyclic amidine . This results in better resistance of the formulation to high relative humidity conditions . Additionally, the dose of saxagliptin should be limited to 2.5 mg once daily when coadministered with strong CYP inhibitors due to increased saxagliptin exposure .
properties
IUPAC Name |
(2S,4S,6R,9S)-9-(3-hydroxy-1-adamantyl)-1,8-diazatricyclo[4.4.0.02,4]decane-7,10-dione |
Source
|
---|---|---|
Source | PubChem | |
URL | https://pubchem.ncbi.nlm.nih.gov | |
Description | Data deposited in or computed by PubChem | |
InChI |
InChI=1S/C18H24N2O3/c21-15-13-3-11-2-12(11)20(13)16(22)14(19-15)17-4-9-1-10(5-17)7-18(23,6-9)8-17/h9-14,23H,1-8H2,(H,19,21)/t9?,10?,11-,12-,13+,14+,17?,18?/m0/s1 |
Source
|
Source | PubChem | |
URL | https://pubchem.ncbi.nlm.nih.gov | |
Description | Data deposited in or computed by PubChem | |
InChI Key |
ZHBXKHDARSBKNT-YGADWWLDSA-N |
Source
|
Source | PubChem | |
URL | https://pubchem.ncbi.nlm.nih.gov | |
Description | Data deposited in or computed by PubChem | |
Canonical SMILES |
C1C2C1N3C(C2)C(=O)NC(C3=O)C45CC6CC(C4)CC(C6)(C5)O |
Source
|
Source | PubChem | |
URL | https://pubchem.ncbi.nlm.nih.gov | |
Description | Data deposited in or computed by PubChem | |
Isomeric SMILES |
C1[C@@H]2[C@H]1N3[C@H](C2)C(=O)N[C@H](C3=O)C45CC6CC(C4)CC(C6)(C5)O |
Source
|
Source | PubChem | |
URL | https://pubchem.ncbi.nlm.nih.gov | |
Description | Data deposited in or computed by PubChem | |
Molecular Formula |
C18H24N2O3 |
Source
|
Source | PubChem | |
URL | https://pubchem.ncbi.nlm.nih.gov | |
Description | Data deposited in or computed by PubChem | |
Molecular Weight |
316.4 g/mol |
Source
|
Source | PubChem | |
URL | https://pubchem.ncbi.nlm.nih.gov | |
Description | Data deposited in or computed by PubChem | |
Product Name |
Saxagliptin Cyclic Amide |
Disclaimer and Information on In-Vitro Research Products
Please be aware that all articles and product information presented on BenchChem are intended solely for informational purposes. The products available for purchase on BenchChem are specifically designed for in-vitro studies, which are conducted outside of living organisms. In-vitro studies, derived from the Latin term "in glass," involve experiments performed in controlled laboratory settings using cells or tissues. It is important to note that these products are not categorized as medicines or drugs, and they have not received approval from the FDA for the prevention, treatment, or cure of any medical condition, ailment, or disease. We must emphasize that any form of bodily introduction of these products into humans or animals is strictly prohibited by law. It is essential to adhere to these guidelines to ensure compliance with legal and ethical standards in research and experimentation.