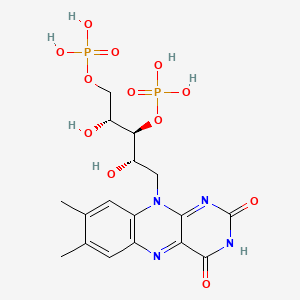
Riboflavin 3',5'-bisphosphate
Overview
Description
Riboflavin 3’,5’-bisphosphate is a derivative of riboflavin, also known as Vitamin B2. This compound is characterized by the presence of two phosphate groups attached to the riboflavin molecule at the 3’ and 5’ positions. Riboflavin itself is an essential nutrient that plays a crucial role in various biochemical processes, including redox reactions, energy production, and cellular function.
Preparation Methods
Synthetic Routes and Reaction Conditions: Riboflavin 3’,5’-bisphosphate can be synthesized through the phosphorylation of riboflavin. The process typically involves the use of phosphorylating agents such as phosphoric acid or phosphorus oxychloride under controlled conditions. The reaction is carried out in an aqueous medium, and the pH is carefully monitored to ensure optimal yield and purity of the product .
Industrial Production Methods: Industrial production of riboflavin 3’,5’-bisphosphate often involves microbial fermentation. Specific strains of bacteria, such as Bacillus subtilis, are genetically engineered to overproduce riboflavin, which is then phosphorylated to form the desired compound. This method is preferred due to its cost-effectiveness and scalability .
Chemical Reactions Analysis
Types of Reactions: Riboflavin 3’,5’-bisphosphate undergoes various chemical reactions, including hydrolysis, rearrangement, and redox reactions. The hydrolysis of the phosphate groups can occur under acidic or basic conditions, leading to the formation of riboflavin monophosphates and free riboflavin .
Common Reagents and Conditions:
Hydrolysis: Acidic or basic conditions, typically using hydrochloric acid or sodium hydroxide.
Rearrangement: Acid-catalyzed isomerization reactions, often conducted at elevated temperatures (50-80°C) and low pH (around 1).
Major Products: The major products formed from these reactions include various isomeric forms of riboflavin monophosphates and free riboflavin .
Scientific Research Applications
Riboflavin 3’,5’-bisphosphate has a wide range of applications in scientific research:
Chemistry: Used as a precursor in the synthesis of flavin derivatives and as a reagent in redox reactions.
Biology: Serves as a cofactor for various enzymes involved in metabolic pathways.
Medicine: Investigated for its potential therapeutic effects, including its role in treating riboflavin deficiency and its use in phototherapy for neonatal jaundice.
Industry: Utilized in the production of food additives, dietary supplements, and pharmaceuticals
Mechanism of Action
Riboflavin 3’,5’-bisphosphate exerts its effects primarily through its role as a precursor to flavin mononucleotide and flavin adenine dinucleotide. These flavin cofactors are essential for the activity of various flavoproteins involved in redox reactions, energy production, and cellular respiration. The compound binds to specific enzymes, facilitating electron transfer and catalyzing biochemical reactions .
Comparison with Similar Compounds
Riboflavin monophosphate: Contains a single phosphate group and is involved in similar biochemical processes.
Flavin mononucleotide: A phosphorylated derivative of riboflavin that serves as a cofactor for various enzymes.
Flavin adenine dinucleotide: A more complex flavin derivative that plays a crucial role in cellular respiration and energy production.
Uniqueness: Riboflavin 3’,5’-bisphosphate is unique due to the presence of two phosphate groups, which enhances its solubility and reactivity compared to other riboflavin derivatives. This makes it particularly useful in specific biochemical and industrial applications .
Properties
IUPAC Name |
[(2S,3S,4R)-1-(7,8-dimethyl-2,4-dioxobenzo[g]pteridin-10-yl)-2,4-dihydroxy-5-phosphonooxypentan-3-yl] dihydrogen phosphate | |
---|---|---|
Source | PubChem | |
URL | https://pubchem.ncbi.nlm.nih.gov | |
Description | Data deposited in or computed by PubChem | |
InChI |
InChI=1S/C17H22N4O12P2/c1-7-3-9-10(4-8(7)2)21(15-13(18-9)16(24)20-17(25)19-15)5-11(22)14(33-35(29,30)31)12(23)6-32-34(26,27)28/h3-4,11-12,14,22-23H,5-6H2,1-2H3,(H,20,24,25)(H2,26,27,28)(H2,29,30,31)/t11-,12+,14-/m0/s1 | |
Source | PubChem | |
URL | https://pubchem.ncbi.nlm.nih.gov | |
Description | Data deposited in or computed by PubChem | |
InChI Key |
JWNUFFDVQFYWGM-SCRDCRAPSA-N | |
Source | PubChem | |
URL | https://pubchem.ncbi.nlm.nih.gov | |
Description | Data deposited in or computed by PubChem | |
Canonical SMILES |
CC1=CC2=C(C=C1C)N(C3=NC(=O)NC(=O)C3=N2)CC(C(C(COP(=O)(O)O)O)OP(=O)(O)O)O | |
Source | PubChem | |
URL | https://pubchem.ncbi.nlm.nih.gov | |
Description | Data deposited in or computed by PubChem | |
Isomeric SMILES |
CC1=CC2=C(C=C1C)N(C3=NC(=O)NC(=O)C3=N2)C[C@@H]([C@@H]([C@@H](COP(=O)(O)O)O)OP(=O)(O)O)O | |
Source | PubChem | |
URL | https://pubchem.ncbi.nlm.nih.gov | |
Description | Data deposited in or computed by PubChem | |
Molecular Formula |
C17H22N4O12P2 | |
Source | PubChem | |
URL | https://pubchem.ncbi.nlm.nih.gov | |
Description | Data deposited in or computed by PubChem | |
Molecular Weight |
536.3 g/mol | |
Source | PubChem | |
URL | https://pubchem.ncbi.nlm.nih.gov | |
Description | Data deposited in or computed by PubChem | |
CAS No. |
86108-26-1 | |
Record name | Riboflavin 3',5'-bisphosphate | |
Source | ChemIDplus | |
URL | https://pubchem.ncbi.nlm.nih.gov/substance/?source=chemidplus&sourceid=0086108261 | |
Description | ChemIDplus is a free, web search system that provides access to the structure and nomenclature authority files used for the identification of chemical substances cited in National Library of Medicine (NLM) databases, including the TOXNET system. | |
Record name | RIBOFLAVIN 3',5'-DIPHOSPHATE | |
Source | FDA Global Substance Registration System (GSRS) | |
URL | https://gsrs.ncats.nih.gov/ginas/app/beta/substances/CM6Z0AL536 | |
Description | The FDA Global Substance Registration System (GSRS) enables the efficient and accurate exchange of information on what substances are in regulated products. Instead of relying on names, which vary across regulatory domains, countries, and regions, the GSRS knowledge base makes it possible for substances to be defined by standardized, scientific descriptions. | |
Explanation | Unless otherwise noted, the contents of the FDA website (www.fda.gov), both text and graphics, are not copyrighted. They are in the public domain and may be republished, reprinted and otherwise used freely by anyone without the need to obtain permission from FDA. Credit to the U.S. Food and Drug Administration as the source is appreciated but not required. | |
Q1: How does riboflavin 3',5'-bisphosphate interact with apoflavodoxins compared to the native flavin mononucleotide (FMN)?
A1: this compound binds to apoflavodoxins, albeit with some key differences compared to FMN. Research has shown that the additional 3'-phosphate group in this compound significantly impacts its binding affinity and kinetics. For instance, the dissociation constant (Kd) of the Megasphaera elsdenii apoflavodoxin complex with this compound is about 23 times higher than that with FMN, indicating weaker binding []. Additionally, the association rate constant for the Desulfovibrio vulgaris apoflavodoxin with this compound is 10 times faster compared to the Megasphaera elsdenii apoflavodoxin reaction []. Interestingly, the 3'-phosphate group of the bound this compound appears to be monoanionic or even neutral, unlike the dianionic state of the 5'-phosphate group, and resides close to or on the protein surface [].
Q2: Does the binding of this compound affect the redox potential of apoflavodoxins?
A2: Yes, the introduction of the 3'-phosphate group in this compound does influence the redox potential of apoflavodoxins, though to a lesser extent than initially anticipated []. While the redox potential of the oxidized/semiquinone transition remains similar to that observed with FMN, the semiquinone-hydroquinone transition exhibits a more negative redox potential by approximately 20 mV []. This suggests that the 3'-phosphate group, despite its protonation upon binding, still impacts the electron transfer properties of the flavin within the protein environment.
Q3: What insights have NMR studies provided about the binding of this compound to apoflavodoxins?
A3: Nuclear magnetic resonance (NMR) spectroscopy has been instrumental in elucidating the structural details of this compound binding to apoflavodoxins. 13C and 15N NMR analyses reveal that the interactions between the isoalloxazine ring of this compound and the protein are largely unaffected by the presence of the additional phosphate group []. This suggests that the binding pocket primarily interacts with the isoalloxazine moiety, while the phosphate groups might be involved in interactions with the protein surface or surrounding solvent. Furthermore, 31P NMR confirms the dianionic nature of the bound 5'-phosphate, mimicking FMN, and the distinct ionization state of the 3'-phosphate upon binding [].
Disclaimer and Information on In-Vitro Research Products
Please be aware that all articles and product information presented on BenchChem are intended solely for informational purposes. The products available for purchase on BenchChem are specifically designed for in-vitro studies, which are conducted outside of living organisms. In-vitro studies, derived from the Latin term "in glass," involve experiments performed in controlled laboratory settings using cells or tissues. It is important to note that these products are not categorized as medicines or drugs, and they have not received approval from the FDA for the prevention, treatment, or cure of any medical condition, ailment, or disease. We must emphasize that any form of bodily introduction of these products into humans or animals is strictly prohibited by law. It is essential to adhere to these guidelines to ensure compliance with legal and ethical standards in research and experimentation.