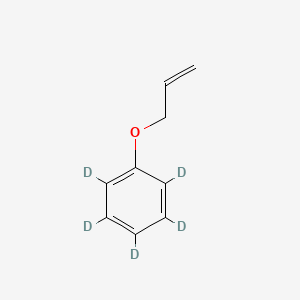
Allyl Phenyl Ether-d5
Overview
Description
Allyl Phenyl Ether-d5: is a deuterated compound with the chemical formula C9H5D5O . It is a colorless liquid with a distinct aromatic odor. The presence of deuterium atoms makes it particularly useful in various scientific research applications, especially in nuclear magnetic resonance (NMR) spectroscopy.
Mechanism of Action
Target of Action
Allyl Phenyl Ether-d5 primarily targets the carbon-oxygen bond in the ether group and the carbon-carbon bond between the allyl group and the phenol ring . The compound’s action is focused on these bonds, leading to significant changes in their structure and connectivity.
Mode of Action
The mode of action of this compound involves a series of steps known as the Claisen rearrangement . This process begins with the breaking of the carbon-oxygen bond, which can occur through a heterolytic cleavage . Following this, a new carbon-carbon bond is formed between the allyl group and the phenol ring . This bond formation occurs after the transition state in the reaction .
Biochemical Pathways
The Claisen rearrangement of this compound affects the electron localization function (ELF) . This rearrangement can be presented as consisting of 10 main steps separated by fold and cusp catastrophes . The reaction leads to the formation of two localized C-C bonds in the phenyl ring, indicating the process of dearomatisation .
Result of Action
The result of the action of this compound is the formation of a new compound, 6-(prop-2-en-1-yl) cyclohexa-2,4-dien-1-one . This compound is formed through the breaking of the C-O bond and the formation of a new C-C bond, along with the migration of a double C-C bond, the formation of a double C-O bond, and the dearomatisation of an aromatic ring .
Action Environment
The action of this compound is influenced by environmental factors such as temperature. For instance, heating an allyl aryl ether to 250°C causes an intramolecular rearrangement to produce an o-allylphenol . Therefore, the compound’s action, efficacy, and stability can be significantly affected by the environment in which it is used.
Preparation Methods
Synthetic Routes and Reaction Conditions: Allyl Phenyl Ether-d5 can be synthesized by the reaction of sodium phenoxide with allyl bromide-d5. The reaction proceeds as follows:
C6H5ONa+BrCD2CD=CD2→C6H5OCD2CD=CD2+NaBr
The reaction is typically conducted in a homogeneous solution using dimethoxyethane, which results in a nearly quantitative yield. When the reaction is conducted as a slurry in diethyl ether, the predominant product is 2-allylphenol after acidic work-up .
Industrial Production Methods: Industrial production of this compound follows similar synthetic routes but on a larger scale. The reaction conditions are optimized to ensure high yield and purity. The use of deuterated reagents and solvents is crucial to maintain the deuterium labeling.
Chemical Reactions Analysis
Types of Reactions:
Claisen Rearrangement: Allyl Phenyl Ether-d5 undergoes Claisen rearrangement in the presence of acid catalysts, converting to 2-allylphenol.
Oxidation and Reduction: The compound can undergo oxidation and reduction reactions, although specific conditions and reagents for these reactions are less commonly reported.
Common Reagents and Conditions:
Acid Catalysts: Used in Claisen rearrangement.
Oxidizing Agents: For potential oxidation reactions.
Reducing Agents: For potential reduction reactions.
Major Products:
2-Allylphenol: Formed through Claisen rearrangement.
Scientific Research Applications
Chemistry:
NMR Spectroscopy: The deuterium atoms in Allyl Phenyl Ether-d5 make it an excellent internal standard for NMR spectroscopy, providing clear and distinct signals.
Biology and Medicine:
Metabolic Studies: Used in metabolic studies to trace the incorporation and transformation of deuterated compounds in biological systems.
Industry:
Polymer Chemistry: Utilized in the synthesis of deuterated polymers for studying polymerization mechanisms and properties.
Comparison with Similar Compounds
Allyl Phenyl Ether: The non-deuterated version of Allyl Phenyl Ether-d5.
Phenyl Vinyl Ether: Another ether compound with similar reactivity.
Allylbenzene: A structurally similar compound with an allyl group attached to a benzene ring.
Uniqueness:
Deuterium Labeling: The presence of deuterium atoms in this compound makes it unique and particularly valuable for NMR spectroscopy and metabolic studies.
Reactivity: While it shares similar reactivity with its non-deuterated counterpart, the deuterium atoms can influence reaction kinetics and mechanisms, providing additional insights in research applications.
Properties
IUPAC Name |
1,2,3,4,5-pentadeuterio-6-prop-2-enoxybenzene | |
---|---|---|
Source | PubChem | |
URL | https://pubchem.ncbi.nlm.nih.gov | |
Description | Data deposited in or computed by PubChem | |
InChI |
InChI=1S/C9H10O/c1-2-8-10-9-6-4-3-5-7-9/h2-7H,1,8H2/i3D,4D,5D,6D,7D | |
Source | PubChem | |
URL | https://pubchem.ncbi.nlm.nih.gov | |
Description | Data deposited in or computed by PubChem | |
InChI Key |
POSICDHOUBKJKP-DKFMXDSJSA-N | |
Source | PubChem | |
URL | https://pubchem.ncbi.nlm.nih.gov | |
Description | Data deposited in or computed by PubChem | |
Canonical SMILES |
C=CCOC1=CC=CC=C1 | |
Source | PubChem | |
URL | https://pubchem.ncbi.nlm.nih.gov | |
Description | Data deposited in or computed by PubChem | |
Isomeric SMILES |
[2H]C1=C(C(=C(C(=C1[2H])[2H])OCC=C)[2H])[2H] | |
Source | PubChem | |
URL | https://pubchem.ncbi.nlm.nih.gov | |
Description | Data deposited in or computed by PubChem | |
Molecular Formula |
C9H10O | |
Source | PubChem | |
URL | https://pubchem.ncbi.nlm.nih.gov | |
Description | Data deposited in or computed by PubChem | |
Molecular Weight |
139.21 g/mol | |
Source | PubChem | |
URL | https://pubchem.ncbi.nlm.nih.gov | |
Description | Data deposited in or computed by PubChem | |
Synthesis routes and methods I
Procedure details
Synthesis routes and methods II
Procedure details
Synthesis routes and methods III
Procedure details
Synthesis routes and methods IV
Procedure details
Synthesis routes and methods V
Procedure details
Retrosynthesis Analysis
AI-Powered Synthesis Planning: Our tool employs the Template_relevance Pistachio, Template_relevance Bkms_metabolic, Template_relevance Pistachio_ringbreaker, Template_relevance Reaxys, Template_relevance Reaxys_biocatalysis model, leveraging a vast database of chemical reactions to predict feasible synthetic routes.
One-Step Synthesis Focus: Specifically designed for one-step synthesis, it provides concise and direct routes for your target compounds, streamlining the synthesis process.
Accurate Predictions: Utilizing the extensive PISTACHIO, BKMS_METABOLIC, PISTACHIO_RINGBREAKER, REAXYS, REAXYS_BIOCATALYSIS database, our tool offers high-accuracy predictions, reflecting the latest in chemical research and data.
Strategy Settings
Precursor scoring | Relevance Heuristic |
---|---|
Min. plausibility | 0.01 |
Model | Template_relevance |
Template Set | Pistachio/Bkms_metabolic/Pistachio_ringbreaker/Reaxys/Reaxys_biocatalysis |
Top-N result to add to graph | 6 |
Feasible Synthetic Routes
Disclaimer and Information on In-Vitro Research Products
Please be aware that all articles and product information presented on BenchChem are intended solely for informational purposes. The products available for purchase on BenchChem are specifically designed for in-vitro studies, which are conducted outside of living organisms. In-vitro studies, derived from the Latin term "in glass," involve experiments performed in controlled laboratory settings using cells or tissues. It is important to note that these products are not categorized as medicines or drugs, and they have not received approval from the FDA for the prevention, treatment, or cure of any medical condition, ailment, or disease. We must emphasize that any form of bodily introduction of these products into humans or animals is strictly prohibited by law. It is essential to adhere to these guidelines to ensure compliance with legal and ethical standards in research and experimentation.