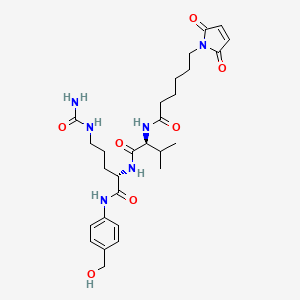
MC-Val-Cit-PAB
Overview
Description
Mechanism of Action
Target of Action
MC-Val-Cit-PAB is a bifunctional cathepsin cleavable linker used in the construction of antibody-drug conjugates (ADCs) . The primary targets of this compound are the cathepsin enzymes present in the lysosomes of cells . These enzymes play a crucial role in protein degradation and turnover, and their activity is particularly high in tumor cells .
Mode of Action
The compound interacts with its targets through a process known as bioconjugation . The maleimide functionality of this compound can be used for bioconjugation to thiols in monoclonal antibodies . The benzyl alcohol part of the compound can be used for the construction of carbamate linkages with amine-containing payloads . This interaction results in the formation of an ADC, which can specifically target and kill tumor cells .
Biochemical Pathways
The biochemical pathway affected by this compound involves the internalization of the ADC into the target cell, followed by the release of the cytotoxic payload within the cell . Once the ADC is internalized, the cathepsin enzymes in the lysosomes cleave the Val-Cit dipeptide motif, releasing the cytotoxic drug . This drug then interacts with its target, often a critical protein or pathway within the cell, leading to cell death .
Pharmacokinetics
The pharmacokinetics of this compound is largely determined by its ADME (Absorption, Distribution, Metabolism, and Excretion) properties . The compound is designed to be stable in the bloodstream, resistant to premature cleavage, and selectively activated within the target cell . This results in a high bioavailability of the cytotoxic drug at the target site, maximizing its therapeutic effect while minimizing off-target toxicity .
Result of Action
The result of the action of this compound is the selective killing of tumor cells . By delivering a cytotoxic drug directly to the target cells, the compound can effectively induce cell death . For example, one study showed that an ADC using this compound demonstrated cytotoxicity with IC50s of 3.3 and 0.95 nM against BJAB and WSU cell lines, respectively .
Action Environment
The action of this compound is influenced by various environmental factors. The presence of cathepsin enzymes in the target cells is crucial for the activation of the compound . Furthermore, the stability and efficacy of the compound can be affected by factors such as pH and the presence of reducing agents . The compound is designed to be stable under physiological conditions, ensuring its effective action in the complex environment of the human body .
Biochemical Analysis
Biochemical Properties
MC-Val-Cit-PAB plays a crucial role in biochemical reactions, particularly in the formation of ADCs . It interacts with enzymes such as cathepsin B, which cleaves the linker in the lysosomes of tumor cells . This interaction is enzymatic and is crucial for the release of the drug payload within the target cells .
Cellular Effects
The effects of this compound on cells are primarily observed through its role in ADCs. Upon internalization of the ADC into the target cell, the this compound linker is cleaved by cathepsin B in the lysosomes, releasing the attached payload inside the target cell . This process influences cell function by delivering potent cytotoxic drugs specifically to the target-expressing cells .
Molecular Mechanism
The molecular mechanism of action of this compound involves two key steps. First, the ADC is internalized into the target cell, where it is transported to the lysosome. Here, the this compound linker is cleaved by cathepsin B, exposing the dipeptide which is then degraded . This degradation liberates the attached payload (drug), allowing it to exert its effects at the molecular level .
Temporal Effects in Laboratory Settings
The stability of this compound in laboratory settings is critical for the efficacy of ADCs. The linker is designed to be stable in circulation but cleavable in the lysosomal environment of the target cell . Over time, the linker remains intact until the ADC is internalized and the linker is cleaved, releasing the drug payload .
Dosage Effects in Animal Models
For instance, ADCs with this compound showed improved tolerability in the hematopoietic compartment in rat studies .
Metabolic Pathways
The metabolic pathway of this compound primarily involves its cleavage by cathepsin B in the lysosomes of target cells . This cleavage is a key step in the metabolic processing of ADCs, leading to the release of the drug payload.
Transport and Distribution
This compound, as part of an ADC, is transported into target cells via receptor-mediated endocytosis . Once inside the cell, the ADC is distributed to the lysosomes, where the this compound linker is cleaved .
Subcellular Localization
The subcellular localization of this compound is within the lysosomes of target cells . Here, the linker is cleaved by cathepsin B, leading to the release of the drug payload . This localization is crucial for the function of ADCs, enabling the targeted delivery of cytotoxic drugs.
Preparation Methods
Synthetic Routes and Reaction Conditions
MC-Val-Cit-PAB is synthesized through a series of chemical reactions involving the coupling of valine-citrulline dipeptide with p-aminobenzyl alcohol. The synthesis typically involves the following steps:
Coupling Reaction: The valine-citrulline dipeptide is coupled with p-aminobenzyl alcohol using a coupling reagent such as EDCI (1-ethyl-3-(3-dimethylaminopropyl)carbodiimide) in the presence of a base like N,N-diisopropylethylamine (DIPEA).
Purification: The crude product is purified using techniques such as column chromatography to obtain the desired this compound compound.
Industrial Production Methods
Industrial production of this compound involves scaling up the synthetic routes mentioned above. The process is optimized for large-scale production by ensuring high yields and purity through controlled reaction conditions and efficient purification methods .
Chemical Reactions Analysis
Types of Reactions
MC-Val-Cit-PAB undergoes several types of chemical reactions, including:
Cleavage Reactions: The compound is designed to be cleaved by cathepsin enzymes in the lysosomes of target cells, releasing the cytotoxic drug.
Substitution Reactions: The maleimide functionality in this compound allows for bioconjugation to thiols in monoclonal antibodies.
Common Reagents and Conditions
Cleavage Reactions: Cathepsin B and related enzymes are commonly used to cleave the valine-citrulline dipeptide motif.
Substitution Reactions: Maleimide and thiol-containing compounds are used for bioconjugation reactions.
Major Products Formed
The major products formed from the cleavage of this compound are the cytotoxic drug and the cleaved linker components. In substitution reactions, the major product is the antibody-drug conjugate .
Scientific Research Applications
MC-Val-Cit-PAB has a wide range of scientific research applications, including:
Comparison with Similar Compounds
MC-Val-Cit-PAB is unique due to its bifunctional nature and cathepsin-cleavable properties. Similar compounds include:
This compound-Cl: Another cleavable ADC linker used for conjugating MMAE and antibodies.
VcMMAE (this compound-MMAE): A compound where this compound is conjugated with MMAE, used in targeted cancer therapies.
This compound stands out due to its high specificity and efficiency in drug delivery, making it a valuable tool in the development of targeted cancer therapies .
Properties
IUPAC Name |
N-[(2S)-1-[[(2S)-5-(carbamoylamino)-1-[4-(hydroxymethyl)anilino]-1-oxopentan-2-yl]amino]-3-methyl-1-oxobutan-2-yl]-6-(2,5-dioxopyrrol-1-yl)hexanamide | |
---|---|---|
Source | PubChem | |
URL | https://pubchem.ncbi.nlm.nih.gov | |
Description | Data deposited in or computed by PubChem | |
InChI |
InChI=1S/C28H40N6O7/c1-18(2)25(33-22(36)8-4-3-5-16-34-23(37)13-14-24(34)38)27(40)32-21(7-6-15-30-28(29)41)26(39)31-20-11-9-19(17-35)10-12-20/h9-14,18,21,25,35H,3-8,15-17H2,1-2H3,(H,31,39)(H,32,40)(H,33,36)(H3,29,30,41)/t21-,25-/m0/s1 | |
Source | PubChem | |
URL | https://pubchem.ncbi.nlm.nih.gov | |
Description | Data deposited in or computed by PubChem | |
InChI Key |
AMKBTTRWLGVRER-OFVILXPXSA-N | |
Source | PubChem | |
URL | https://pubchem.ncbi.nlm.nih.gov | |
Description | Data deposited in or computed by PubChem | |
Canonical SMILES |
CC(C)C(C(=O)NC(CCCNC(=O)N)C(=O)NC1=CC=C(C=C1)CO)NC(=O)CCCCCN2C(=O)C=CC2=O | |
Source | PubChem | |
URL | https://pubchem.ncbi.nlm.nih.gov | |
Description | Data deposited in or computed by PubChem | |
Isomeric SMILES |
CC(C)[C@@H](C(=O)N[C@@H](CCCNC(=O)N)C(=O)NC1=CC=C(C=C1)CO)NC(=O)CCCCCN2C(=O)C=CC2=O | |
Source | PubChem | |
URL | https://pubchem.ncbi.nlm.nih.gov | |
Description | Data deposited in or computed by PubChem | |
Molecular Formula |
C28H40N6O7 | |
Source | PubChem | |
URL | https://pubchem.ncbi.nlm.nih.gov | |
Description | Data deposited in or computed by PubChem | |
DSSTOX Substance ID |
DTXSID201103437 | |
Record name | N-[6-(2,5-Dihydro-2,5-dioxo-1H-pyrrol-1-yl)-1-oxohexyl]-L-valyl-N5-(aminocarbonyl)-N-[4-(hydroxymethyl)phenyl]-L-ornithinamide | |
Source | EPA DSSTox | |
URL | https://comptox.epa.gov/dashboard/DTXSID201103437 | |
Description | DSSTox provides a high quality public chemistry resource for supporting improved predictive toxicology. | |
Molecular Weight |
572.7 g/mol | |
Source | PubChem | |
URL | https://pubchem.ncbi.nlm.nih.gov | |
Description | Data deposited in or computed by PubChem | |
CAS No. |
159857-80-4 | |
Record name | N-[6-(2,5-Dihydro-2,5-dioxo-1H-pyrrol-1-yl)-1-oxohexyl]-L-valyl-N5-(aminocarbonyl)-N-[4-(hydroxymethyl)phenyl]-L-ornithinamide | |
Source | CAS Common Chemistry | |
URL | https://commonchemistry.cas.org/detail?cas_rn=159857-80-4 | |
Description | CAS Common Chemistry is an open community resource for accessing chemical information. Nearly 500,000 chemical substances from CAS REGISTRY cover areas of community interest, including common and frequently regulated chemicals, and those relevant to high school and undergraduate chemistry classes. This chemical information, curated by our expert scientists, is provided in alignment with our mission as a division of the American Chemical Society. | |
Explanation | The data from CAS Common Chemistry is provided under a CC-BY-NC 4.0 license, unless otherwise stated. | |
Record name | N-[6-(2,5-Dihydro-2,5-dioxo-1H-pyrrol-1-yl)-1-oxohexyl]-L-valyl-N5-(aminocarbonyl)-N-[4-(hydroxymethyl)phenyl]-L-ornithinamide | |
Source | EPA DSSTox | |
URL | https://comptox.epa.gov/dashboard/DTXSID201103437 | |
Description | DSSTox provides a high quality public chemistry resource for supporting improved predictive toxicology. | |
Q1: What is the role of MC-Val-Cit-PAB in antibody-drug conjugates?
A: this compound serves as a critical linker in ADCs, acting as a bridge between a monoclonal antibody and a cytotoxic payload [, ]. This linker is designed to be stable in circulation but cleavable within the target cancer cells, allowing for the controlled release of the cytotoxic drug specifically at the tumor site [].
Q2: How does the structure of this compound contribute to its function in ADCs?
A: this compound incorporates a valine-citrulline dipeptide sequence. This sequence is recognized and cleaved by specific proteases found within the lysosomes of tumor cells [, ]. This cleavage mechanism ensures that the cytotoxic drug is released only after the ADC has been internalized by the target cancer cell, minimizing off-target effects [, ].
Q3: Can you provide an example of an ADC utilizing this compound and its application in cancer therapy?
A: One study utilized this compound to create an ADC by conjugating the cytotoxic drug Doxorubicin (DOX) to Cetuximab, an antibody targeting the epidermal growth factor receptor (EGFR) []. This cetuximab-vc-DOX ADC demonstrated promising results in targeting and inhibiting the growth of colorectal cancer cells overexpressing EGFR both in vitro and in vivo []. The researchers highlighted the enhanced tumor targeting ability and reduced systemic toxicity of this ADC, suggesting its potential as a targeted therapy for colorectal cancer [].
Disclaimer and Information on In-Vitro Research Products
Please be aware that all articles and product information presented on BenchChem are intended solely for informational purposes. The products available for purchase on BenchChem are specifically designed for in-vitro studies, which are conducted outside of living organisms. In-vitro studies, derived from the Latin term "in glass," involve experiments performed in controlled laboratory settings using cells or tissues. It is important to note that these products are not categorized as medicines or drugs, and they have not received approval from the FDA for the prevention, treatment, or cure of any medical condition, ailment, or disease. We must emphasize that any form of bodily introduction of these products into humans or animals is strictly prohibited by law. It is essential to adhere to these guidelines to ensure compliance with legal and ethical standards in research and experimentation.