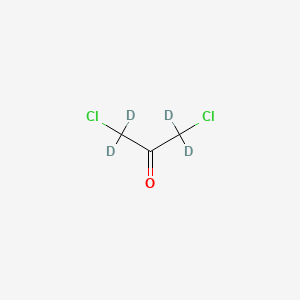
1,3-Dichloroacetone-D4
Overview
Description
1,3-Dichloroacetone-D4 is a deuterated form of 1,3-dichloroacetone, a dihaloketone derivative. This compound is characterized by the presence of two chlorine atoms attached to the first and third carbon atoms of the acetone molecule, with deuterium atoms replacing the hydrogen atoms. It is a crystalline solid with a melting point of 39-41°C and is known for its high chemical reactivity due to the presence of three vicinal electrophilic carbons .
Preparation Methods
Synthetic Routes and Reaction Conditions
1,3-Dichloroacetone-D4 can be synthesized through the chlorination of deuterated acetone in methanol. The reaction involves the substitution of hydrogen atoms with chlorine atoms in the presence of a chlorinating agent. An alternative method involves the in situ formation of a lithium carbenoid, such as chloromethyllithium, which reacts with the ethyl ester of deuterated chloroacetic acid .
Industrial Production Methods
Industrial production of this compound typically follows the same synthetic routes as laboratory methods but on a larger scale. The process involves the careful control of reaction conditions to ensure high yield and purity of the final product. The use of advanced purification techniques, such as distillation and recrystallization, is common to achieve the desired chemical purity .
Chemical Reactions Analysis
Types of Reactions
1,3-Dichloroacetone-D4 undergoes various chemical reactions, including:
Substitution Reactions: The chlorine atoms can be substituted by nucleophiles such as oxygen, sulfur, and nitrogen-containing compounds.
Addition Reactions: The compound can participate in addition reactions with nucleophiles, leading to the formation of new carbon-carbon or carbon-heteroatom bonds.
Elimination Reactions: Under certain conditions, this compound can undergo elimination reactions to form unsaturated compounds
Common Reagents and Conditions
Nucleophiles: Oxygen, sulfur, and nitrogen-containing nucleophiles are commonly used in substitution reactions.
Catalysts: Catalysts such as copper(II) and palladium are often employed to facilitate the reactions.
Solvents: Organic solvents like tetrahydrofuran (THF) and methanol are frequently used to dissolve the reactants and control the reaction environment
Major Products
The major products formed from these reactions include substituted acetones, unsaturated ketones, and various cyclic compounds. The specific products depend on the nature of the nucleophile and the reaction conditions .
Scientific Research Applications
Chemical Synthesis
1,3-Dichloroacetone-D4 serves as an important reagent in organic synthesis. Its unique structure allows it to participate in various chemical reactions, making it a valuable tool for chemists.
Key Applications:
- Synthesis of Bicyclic Peptides : Research indicates that 1,3-dichloroacetone can be used to prepare bicyclic peptides through selective linking of cysteine side chains. This method utilizes an S_N2 reaction to create stable thioether bridges between cyclic peptide monomers. The resulting dimeric peptides exhibit enhanced stability and potential therapeutic applications .
- Cross-Linking Agent : In protein chemistry, this compound is utilized as a cross-linking agent to stabilize protein structures. It forms dithioether linkages that can influence protein folding and function, thereby aiding in the study of protein interactions and dynamics .
Biochemical Applications
The biochemical applications of this compound extend to the fields of proteomics and drug development.
Case Studies:
- Protein Labeling : A study demonstrated the use of 1,3-dichloroacetone for selective labeling of proteins. This technique allows researchers to probe protein functions in vitro and in living systems, providing insights into cellular processes and potential therapeutic targets .
- Peptide Macrocyclization : The compound has been employed in the macrocyclization of peptides, facilitating the formation of stable cyclic structures that can enhance biological activity. This application is particularly relevant in drug design, where cyclic peptides often exhibit improved pharmacological properties compared to their linear counterparts .
Data Tables
The following tables summarize key findings related to the applications of this compound:
Table 1: Reaction Conditions for Dimerization of Cyclic Peptides
Condition | Dimerization Yield (%) | Reaction Time (h) | Notes |
---|---|---|---|
Condition 1 | 67 | 1 | Baseline condition |
Condition 2 | 77 | 1 | Improved yield with DMF as cosolvent |
Table 2: Protein Cross-Linking Comparisons
Cross-Linking Method | Type of Linkage | Stability Observed (h) | Application |
---|---|---|---|
Disulfide Bond | Covalent | Variable | General protein stabilization |
Dithioether (DCA) | Covalent | >48 | Enhanced structural integrity |
Mechanism of Action
The mechanism of action of 1,3-dichloroacetone-D4 involves its high reactivity due to the presence of electrophilic carbons. The compound can readily undergo nucleophilic substitution reactions, where nucleophiles attack the electrophilic carbon atoms, leading to the formation of new bonds. This reactivity makes it a valuable tool in organic synthesis, particularly in the formation of complex cyclic structures .
Comparison with Similar Compounds
Similar Compounds
1,3-Dichloroacetone: The non-deuterated form of the compound, which has similar reactivity but different isotopic composition.
1,3-Dichloro-2-propanol: A related compound with a hydroxyl group instead of a carbonyl group.
1,3-Dibromoacetone: A similar compound with bromine atoms instead of chlorine atoms.
1,3-Difluoroacetone: A compound with fluorine atoms instead of chlorine atoms.
Uniqueness
1,3-Dichloroacetone-D4 is unique due to its deuterated nature, which makes it useful in studies involving isotopic labeling. The presence of deuterium atoms can influence the compound’s physical and chemical properties, making it valuable in various research applications, including mechanistic studies and the development of deuterium-labeled drugs .
Biological Activity
1,3-Dichloroacetone-D4 (DCA-D4) is a deuterated derivative of 1,3-dichloroacetone, a compound known for its utility in organic synthesis and biological research. This article explores the biological activity of DCA-D4, focusing on its mechanism of action, biochemical pathways, cellular effects, and applications in scientific research.
Target of Action
DCA-D4 primarily targets free cysteine side-chains in proteins. This interaction is facilitated through an SN2 reaction , which allows DCA-D4 to selectively link these side chains, leading to the formation of complex peptide structures.
Mode of Action
The SN2 reaction mediated by DCA-D4 is crucial for synthesizing bicyclic dimeric peptides , which are significant for various biochemical applications. This reaction not only enhances the structural complexity of peptides but also influences their stability and biological activity .
Biochemical Pathways
DCA-D4 plays a vital role in several biochemical pathways:
- Peptide Dimerization : The compound's ability to facilitate peptide dimerization is instrumental in creating multicyclic peptides that can modulate biological processes such as cell signaling and gene expression.
- Proteolytic Stability : Studies have shown that peptides synthesized using DCA-D4 maintain their integrity in human serum for extended periods (e.g., fully intact after 48 hours), indicating potential therapeutic applications .
Cellular Effects
The effects of DCA-D4 on cellular processes are primarily linked to its role in peptide synthesis:
- Cell Signaling : The formation of bicyclic peptides can influence various signaling pathways, potentially impacting cellular responses to external stimuli.
- Gene Expression : By altering peptide structures, DCA-D4 may affect transcription factors and other regulatory proteins involved in gene expression.
Table 1: Summary of Key Research Findings on DCA-D4
Case Study: Synthesis of Bicyclic Peptides
In a study aimed at synthesizing bicyclic peptides using DCA-D4, researchers designed cyclic peptide monomers based on neurotrophin sequences. The study identified optimal reaction conditions that yielded high dimerization rates while ensuring that alternative nucleophiles, such as lysine amines, did not interfere with the desired reactions .
The resulting bicyclic peptides demonstrated enhanced metabolic stability compared to traditional cyclic peptides, suggesting their potential as pharmacological tools or lead compounds for further development .
Applications in Scientific Research
DCA-D4 has several notable applications across different fields:
- Chemistry : Utilized as a reagent for synthesizing complex multicyclic peptides.
- Biology : Employed in studies related to enzyme-catalyzed reactions and metabolic pathways.
- Medicine : Investigated for its role in drug discovery, particularly in developing peptide-based therapeutics.
- Industry : Used as an intermediate in the production of fine chemicals .
Q & A
Basic Research Questions
Q. What are the key considerations for synthesizing 1,3-Dichloroacetone-D4 with high isotopic purity?
Answer:
Deuterated compounds like this compound require precise control over reaction conditions to ensure isotopic purity. Key steps include:
- Deuterium Source Selection : Use deuterated solvents (e.g., D₂O) or reagents to minimize proton contamination.
- Reaction Optimization : Monitor reaction kinetics to avoid isotopic scrambling, which can occur under prolonged heating or acidic/basic conditions.
- Purification : Employ fractional distillation or chromatography to isolate the deuterated product. Validate purity via mass spectrometry (MS) to confirm the absence of non-deuterated analogs (e.g., m/z 126.98 for non-deuterated 1,3-Dichloroacetone vs. m/z 130.99 for the deuterated form) .
- Storage : Store at 0–6°C to prevent degradation, as indicated by its cold storage requirements .
Q. How is this compound characterized using spectroscopic techniques?
Answer:
Characterization involves a combination of techniques:
- NMR Spectroscopy :
- ¹H NMR : Signals for residual protons in the deuterated compound should be minimal. The methyl groups (CD₂) typically show no peaks in the proton spectrum.
- ¹³C NMR : Peaks for carbonyl (C=O) and chlorinated carbons (C-Cl) should align with theoretical shifts (~200 ppm for C=O, ~50 ppm for C-Cl).
- Mass Spectrometry : High-resolution MS (HRMS) confirms molecular ion clusters (m/z 130.99) and isotopic patterns consistent with D₄ labeling .
- IR Spectroscopy : Verify C=O stretching (~1700 cm⁻¹) and C-Cl bonds (~600 cm⁻¹).
Technique | Key Peaks/Data |
---|---|
¹H NMR | No residual H signals |
HRMS | m/z 130.99 (M⁺) |
IR | 1700 cm⁻¹ (C=O) |
Q. What safety protocols are critical when handling this compound?
Answer:
- Personal Protective Equipment (PPE) : Use nitrile gloves, lab coats, and safety goggles to avoid skin/eye contact.
- Ventilation : Work in a fume hood due to potential volatility and respiratory hazards .
- Spill Management : Neutralize spills with inert adsorbents (e.g., vermiculite) and dispose of as hazardous waste.
- First Aid : For inhalation, move to fresh air; for skin contact, wash with soap and water .
Q. How is this compound used as an internal standard in environmental analyses?
Answer:
Its deuterated structure minimizes interference with non-deuterated analytes in techniques like GC-MS:
- Sample Preparation : Spike samples with a known concentration of this compound to correct for matrix effects.
- Quantification : Use the deuterated compound’s signal to normalize analyte responses, improving accuracy in trace analysis (e.g., pesticide residues) .
Q. What are the storage requirements for this compound to ensure stability?
Answer:
- Temperature : Store at 0–6°C to prevent thermal degradation .
- Container : Use amber glass vials to avoid light-induced decomposition.
- Inert Atmosphere : Seal under nitrogen or argon to reduce oxidation .
Advanced Research Questions
Q. How does deuteration affect the reactivity of this compound in kinetic studies compared to its non-deuterated analog?
Answer:
Deuteration introduces kinetic isotope effects (KIEs), altering reaction rates:
- Mechanistic Insights : Use comparative studies to probe reaction pathways. For example, slower hydrolysis rates in deuterated compounds may indicate proton transfer as a rate-limiting step.
- Experimental Design : Conduct parallel reactions with deuterated and non-deuterated forms under identical conditions. Analyze rate constants via Arrhenius plots .
Q. What methodologies resolve data discrepancies when using this compound in multi-analyte environmental studies?
Answer:
- Cross-Validation : Compare results across multiple analytical platforms (e.g., GC-MS vs. LC-MS/MS).
- Matrix Spike Recovery Tests : Assess interference from co-eluting compounds.
- Statistical Analysis : Apply multivariate regression to isolate confounding variables (e.g., pH, temperature) .
Q. How can this compound be integrated into studies of halogenated acetone derivatives’ environmental fate?
Answer:
- Tracer Experiments : Use this compound to track degradation pathways (e.g., photolysis, microbial metabolism) in soil/water systems.
- Isotopic Labeling : Monitor deuterium retention to distinguish biotic vs. abiotic degradation mechanisms .
Q. What strategies optimize the detection limits of this compound in trace analysis?
Answer:
- Derivatization : Enhance volatility via silylation or acylation for GC-MS applications.
- Preconcentration : Use solid-phase microextraction (SPME) to isolate the compound from complex matrices.
- Instrument Tuning : Optimize MS parameters (e.g., collision energy) to maximize ion yield .
Q. How should literature reviews on halogenated acetone derivatives be structured to identify research gaps?
Answer:
- Search Strategy : Use databases (PubMed, TOXLINE) with keywords: “deuterated acetone derivatives,” “chlorinated acetone environmental impact,” and CAS-specific terms (e.g., 350818-52-9) .
- Critical Appraisal : Prioritize peer-reviewed studies with robust methodologies (e.g., controlled variables, replicate analyses). Synthesize findings into tables highlighting contradictions (e.g., conflicting degradation half-lives) .
Properties
IUPAC Name |
1,3-dichloro-1,1,3,3-tetradeuteriopropan-2-one | |
---|---|---|
Source | PubChem | |
URL | https://pubchem.ncbi.nlm.nih.gov | |
Description | Data deposited in or computed by PubChem | |
InChI |
InChI=1S/C3H4Cl2O/c4-1-3(6)2-5/h1-2H2/i1D2,2D2 | |
Source | PubChem | |
URL | https://pubchem.ncbi.nlm.nih.gov | |
Description | Data deposited in or computed by PubChem | |
InChI Key |
SUNMBRGCANLOEG-LNLMKGTHSA-N | |
Source | PubChem | |
URL | https://pubchem.ncbi.nlm.nih.gov | |
Description | Data deposited in or computed by PubChem | |
Canonical SMILES |
C(C(=O)CCl)Cl | |
Source | PubChem | |
URL | https://pubchem.ncbi.nlm.nih.gov | |
Description | Data deposited in or computed by PubChem | |
Isomeric SMILES |
[2H]C([2H])(C(=O)C([2H])([2H])Cl)Cl | |
Source | PubChem | |
URL | https://pubchem.ncbi.nlm.nih.gov | |
Description | Data deposited in or computed by PubChem | |
Molecular Formula |
C3H4Cl2O | |
Source | PubChem | |
URL | https://pubchem.ncbi.nlm.nih.gov | |
Description | Data deposited in or computed by PubChem | |
Molecular Weight |
130.99 g/mol | |
Source | PubChem | |
URL | https://pubchem.ncbi.nlm.nih.gov | |
Description | Data deposited in or computed by PubChem | |
Disclaimer and Information on In-Vitro Research Products
Please be aware that all articles and product information presented on BenchChem are intended solely for informational purposes. The products available for purchase on BenchChem are specifically designed for in-vitro studies, which are conducted outside of living organisms. In-vitro studies, derived from the Latin term "in glass," involve experiments performed in controlled laboratory settings using cells or tissues. It is important to note that these products are not categorized as medicines or drugs, and they have not received approval from the FDA for the prevention, treatment, or cure of any medical condition, ailment, or disease. We must emphasize that any form of bodily introduction of these products into humans or animals is strictly prohibited by law. It is essential to adhere to these guidelines to ensure compliance with legal and ethical standards in research and experimentation.