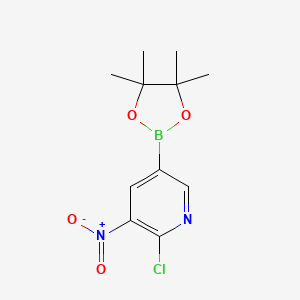
2-Chloro-3-nitro-5-(4,4,5,5-tetramethyl-1,3,2-dioxaborolan-2-YL)pyridine
Overview
Description
2-Chloro-3-nitro-5-(4,4,5,5-tetramethyl-1,3,2-dioxaborolan-2-YL)pyridine: is a complex organic compound that belongs to the class of boronic esters. This compound is characterized by the presence of a pyridine ring substituted with chloro, nitro, and boronic ester groups. It is widely used in organic synthesis, particularly in cross-coupling reactions, due to its unique reactivity and stability.
Preparation Methods
Synthetic Routes and Reaction Conditions
The synthesis of 2-Chloro-3-nitro-5-(4,4,5,5-tetramethyl-1,3,2-dioxaborolan-2-YL)pyridine typically involves the following steps:
Nitration: The starting material, 2-chloropyridine, undergoes nitration using a mixture of concentrated nitric acid and sulfuric acid to yield 2-chloro-3-nitropyridine.
Borylation: The nitrated product is then subjected to a borylation reaction. This involves the use of a boronic ester reagent, such as bis(pinacolato)diboron, in the presence of a palladium catalyst and a base like potassium carbonate. The reaction is typically carried out in an inert atmosphere, such as nitrogen or argon, at elevated temperatures.
Industrial Production Methods
In an industrial setting, the production of this compound follows similar synthetic routes but on a larger scale. The process involves:
Batch or Continuous Flow Reactors: These reactors are used to handle large volumes of reactants and ensure efficient mixing and heat transfer.
Catalyst Recovery and Recycling: Palladium catalysts are often recovered and recycled to minimize costs and environmental impact.
Purification: The final product is purified using techniques such as crystallization, distillation, or chromatography to achieve the desired purity.
Chemical Reactions Analysis
Types of Reactions
2-Chloro-3-nitro-5-(4,4,5,5-tetramethyl-1,3,2-dioxaborolan-2-YL)pyridine undergoes various chemical reactions, including:
Substitution Reactions: The chloro and nitro groups can be substituted with other functional groups through nucleophilic or electrophilic substitution reactions.
Cross-Coupling Reactions: The boronic ester group participates in Suzuki-Miyaura cross-coupling reactions, forming carbon-carbon bonds with aryl or vinyl halides.
Reduction Reactions: The nitro group can be reduced to an amino group using reducing agents such as hydrogen gas in the presence of a palladium catalyst.
Common Reagents and Conditions
Palladium Catalysts: Used in cross-coupling and reduction reactions.
Bases: Potassium carbonate or sodium hydroxide are commonly used bases.
Solvents: Organic solvents like tetrahydrofuran (THF), dimethylformamide (DMF), or ethanol are typically used.
Major Products
Aminopyridine Derivatives: Formed through the reduction of the nitro group.
Biaryl Compounds: Produced via Suzuki-Miyaura cross-coupling reactions.
Scientific Research Applications
2-Chloro-3-nitro-5-(4,4,5,5-tetramethyl-1,3,2-dioxaborolan-2-YL)pyridine has several applications in scientific research:
Organic Synthesis: Used as a building block in the synthesis of complex organic molecules.
Medicinal Chemistry: Employed in the development of pharmaceuticals, particularly in the synthesis of biologically active compounds.
Material Science: Utilized in the preparation of advanced materials, such as polymers and nanomaterials.
Chemical Biology: Applied in the study of biological systems and the development of chemical probes.
Mechanism of Action
The mechanism of action of 2-Chloro-3-nitro-5-(4,4,5,5-tetramethyl-1,3,2-dioxaborolan-2-YL)pyridine primarily involves its role as a reagent in chemical reactions. The boronic ester group facilitates cross-coupling reactions by forming a transient boronate complex with the palladium catalyst. This complex then undergoes transmetallation with an aryl or vinyl halide, followed by reductive elimination to form the desired carbon-carbon bond.
Comparison with Similar Compounds
Similar Compounds
2-Chloro-3-nitropyridine: Lacks the boronic ester group, making it less versatile in cross-coupling reactions.
4,4,5,5-Tetramethyl-1,3,2-dioxaborolane: A simpler boronic ester without the pyridine ring, used in similar borylation reactions.
2-Nitro-5-pyridineboronic acid pinacol ester: Similar structure but with different substitution patterns on the pyridine ring.
Uniqueness
The uniqueness of 2-Chloro-3-nitro-5-(4,4,5,5-tetramethyl-1,3,2-dioxaborolan-2-YL)pyridine lies in its combination of functional groups, which allows it to participate in a wide range of chemical reactions. Its boronic ester group makes it particularly valuable in Suzuki-Miyaura cross-coupling reactions, while the chloro and nitro groups provide additional sites for further functionalization.
Biological Activity
2-Chloro-3-nitro-5-(4,4,5,5-tetramethyl-1,3,2-dioxaborolan-2-YL)pyridine (CAS: 1310383-11-9) is a compound of interest in medicinal chemistry due to its potential biological activities. This article provides a comprehensive overview of its biological activity, including synthesis, mechanisms of action, and relevant case studies.
- Molecular Formula : C11H14BClN2O4
- Molecular Weight : 284.5 g/mol
- Physical State : Solid
- Purity : ≥ 96% .
The compound's biological activity is primarily attributed to its structural components. The presence of the nitro group and the dioxaborolane moiety suggests potential interactions with biological targets such as enzymes and receptors. Its mechanism may involve:
- Inhibition of Enzymatic Activity : Potential inhibition of specific enzymes related to cancer proliferation.
- Interaction with Cellular Pathways : Modulation of signaling pathways that regulate cell growth and apoptosis.
Biological Activity Overview
Research indicates that this compound exhibits a range of biological activities:
Anticancer Activity
Several studies have explored the anticancer properties of this compound:
- Cell Proliferation Inhibition : In vitro assays demonstrated significant inhibition of cancer cell lines with IC50 values ranging from 0.1 µM to 10 µM depending on the specific cell line and treatment duration.
- Mechanism Studies : The compound was found to induce apoptosis in cancer cells through caspase activation and disruption of mitochondrial membrane potential.
Table 1: Summary of Anticancer Activity
Cell Line | IC50 (µM) | Mechanism of Action |
---|---|---|
A549 (Lung) | 0.56 | Apoptosis via caspase activation |
MDA-MB-231 (Breast) | 0.23 | Inhibition of tubulin polymerization |
HL-60 (Leukemia) | 1.0 | Cell cycle arrest |
Study 1: Antiproliferative Effects on Lung Cancer Cells
A study conducted on A549 lung cancer cells revealed that treatment with the compound resulted in a significant decrease in cell viability after 24 hours, with an IC50 value of 0.56 µM. Flow cytometry analysis indicated an increase in apoptotic cells compared to the control group.
Study 2: Mechanistic Insights into Tumor Suppression
In another investigation focusing on MDA-MB-231 breast cancer cells, the compound was shown to inhibit tubulin polymerization effectively. This was evidenced by an IC50 value of 0.23 µM, suggesting that it may disrupt mitotic spindle formation during cell division.
Pharmacokinetics and Toxicology
Understanding the pharmacokinetics and potential toxicity is crucial for evaluating the therapeutic viability of this compound:
- Absorption and Distribution : Preliminary studies indicate moderate permeability across cellular membranes.
- Metabolism : The compound undergoes hepatic metabolism; however, specific metabolic pathways remain to be elucidated.
- Toxicity Profile : Acute toxicity studies suggest that while the compound exhibits anticancer properties, it also poses risks at higher concentrations.
Properties
IUPAC Name |
2-chloro-3-nitro-5-(4,4,5,5-tetramethyl-1,3,2-dioxaborolan-2-yl)pyridine | |
---|---|---|
Source | PubChem | |
URL | https://pubchem.ncbi.nlm.nih.gov | |
Description | Data deposited in or computed by PubChem | |
InChI |
InChI=1S/C11H14BClN2O4/c1-10(2)11(3,4)19-12(18-10)7-5-8(15(16)17)9(13)14-6-7/h5-6H,1-4H3 | |
Source | PubChem | |
URL | https://pubchem.ncbi.nlm.nih.gov | |
Description | Data deposited in or computed by PubChem | |
InChI Key |
CUGHXJSXZTYJEK-UHFFFAOYSA-N | |
Source | PubChem | |
URL | https://pubchem.ncbi.nlm.nih.gov | |
Description | Data deposited in or computed by PubChem | |
Canonical SMILES |
B1(OC(C(O1)(C)C)(C)C)C2=CC(=C(N=C2)Cl)[N+](=O)[O-] | |
Source | PubChem | |
URL | https://pubchem.ncbi.nlm.nih.gov | |
Description | Data deposited in or computed by PubChem | |
Molecular Formula |
C11H14BClN2O4 | |
Source | PubChem | |
URL | https://pubchem.ncbi.nlm.nih.gov | |
Description | Data deposited in or computed by PubChem | |
DSSTOX Substance ID |
DTXSID801144013 | |
Record name | Pyridine, 2-chloro-3-nitro-5-(4,4,5,5-tetramethyl-1,3,2-dioxaborolan-2-yl)- | |
Source | EPA DSSTox | |
URL | https://comptox.epa.gov/dashboard/DTXSID801144013 | |
Description | DSSTox provides a high quality public chemistry resource for supporting improved predictive toxicology. | |
Molecular Weight |
284.50 g/mol | |
Source | PubChem | |
URL | https://pubchem.ncbi.nlm.nih.gov | |
Description | Data deposited in or computed by PubChem | |
CAS No. |
1310383-11-9 | |
Record name | Pyridine, 2-chloro-3-nitro-5-(4,4,5,5-tetramethyl-1,3,2-dioxaborolan-2-yl)- | |
Source | CAS Common Chemistry | |
URL | https://commonchemistry.cas.org/detail?cas_rn=1310383-11-9 | |
Description | CAS Common Chemistry is an open community resource for accessing chemical information. Nearly 500,000 chemical substances from CAS REGISTRY cover areas of community interest, including common and frequently regulated chemicals, and those relevant to high school and undergraduate chemistry classes. This chemical information, curated by our expert scientists, is provided in alignment with our mission as a division of the American Chemical Society. | |
Explanation | The data from CAS Common Chemistry is provided under a CC-BY-NC 4.0 license, unless otherwise stated. | |
Record name | Pyridine, 2-chloro-3-nitro-5-(4,4,5,5-tetramethyl-1,3,2-dioxaborolan-2-yl)- | |
Source | EPA DSSTox | |
URL | https://comptox.epa.gov/dashboard/DTXSID801144013 | |
Description | DSSTox provides a high quality public chemistry resource for supporting improved predictive toxicology. | |
Disclaimer and Information on In-Vitro Research Products
Please be aware that all articles and product information presented on BenchChem are intended solely for informational purposes. The products available for purchase on BenchChem are specifically designed for in-vitro studies, which are conducted outside of living organisms. In-vitro studies, derived from the Latin term "in glass," involve experiments performed in controlled laboratory settings using cells or tissues. It is important to note that these products are not categorized as medicines or drugs, and they have not received approval from the FDA for the prevention, treatment, or cure of any medical condition, ailment, or disease. We must emphasize that any form of bodily introduction of these products into humans or animals is strictly prohibited by law. It is essential to adhere to these guidelines to ensure compliance with legal and ethical standards in research and experimentation.