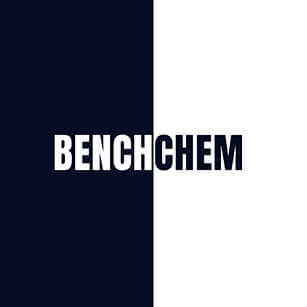
1,2,3,4-Butanetetracarboxylic acid polymer with 2,2-bis(hydroxymethyl)-1,3-propane- diol and 3-hydro
- Click on QUICK INQUIRY to receive a quote from our team of experts.
- With the quality product at a COMPETITIVE price, you can focus more on your research.
Overview
Description
1,2,3,4-Butanetetracarboxylic acid polymer with 2,2-bis(hydroxymethyl)-1,3-propane-diol and 3-hydro is a complex polymeric compound. It is primarily used in various industrial applications due to its unique chemical properties, including its ability to form stable, cross-linked networks. This compound is particularly valued in the textile industry for its role in fabric finishing, providing enhanced durability and resistance to wrinkling.
Preparation Methods
Synthetic Routes and Reaction Conditions
The synthesis of 1,2,3,4-Butanetetracarboxylic acid polymer with 2,2-bis(hydroxymethyl)-1,3-propane-diol and 3-hydro typically involves a polycondensation reaction. The process begins with the esterification of 1,2,3,4-butanetetracarboxylic acid with 2,2-bis(hydroxymethyl)-1,3-propane-diol. This reaction is carried out under acidic conditions, often using a catalyst such as sulfuric acid to facilitate the reaction. The reaction mixture is heated to a temperature range of 150-200°C to promote the formation of ester bonds.
Industrial Production Methods
In an industrial setting, the production of this polymer involves large-scale reactors where the reactants are continuously fed and the reaction conditions are meticulously controlled. The polymerization process is monitored to ensure the desired molecular weight and degree of polymerization are achieved. Post-reaction, the polymer is purified and processed into the desired form, whether it be a powder, solution, or film.
Chemical Reactions Analysis
Types of Reactions
1,2,3,4-Butanetetracarboxylic acid polymer with 2,2-bis(hydroxymethyl)-1,3-propane-diol and 3-hydro undergoes various chemical reactions, including:
Esterification: As mentioned, the initial formation involves esterification.
Cross-linking: The polymer can undergo cross-linking reactions, which are crucial for its application in textile finishing.
Hydrolysis: Under certain conditions, the ester bonds in the polymer can be hydrolyzed, leading to the breakdown of the polymer.
Common Reagents and Conditions
Catalysts: Sulfuric acid or other strong acids for esterification.
Cross-linking Agents: Formaldehyde or other aldehydes can be used to induce cross-linking.
Hydrolysis Conditions: Acidic or basic conditions can promote hydrolysis.
Major Products
Cross-linked Polymers: These are the primary products when cross-linking agents are used.
Monomeric Units: Hydrolysis can yield the original monomeric units, such as 1,2,3,4-butanetetracarboxylic acid and 2,2-bis(hydroxymethyl)-1,3-propane-diol.
Scientific Research Applications
1,2,3,4-Butanetetracarboxylic acid polymer with 2,2-bis(hydroxymethyl)-1,3-propane-diol and 3-hydro has several applications in scientific research:
Textile Industry: Used as a finishing agent to improve fabric durability and resistance to wrinkling.
Biomedical Applications: Potential use in drug delivery systems due to its ability to form stable, biocompatible networks.
Material Science: Research into its use as a precursor for advanced materials with specific mechanical and chemical properties.
Mechanism of Action
The primary mechanism by which this polymer exerts its effects is through the formation of stable, cross-linked networks. These networks enhance the mechanical properties of the materials they are applied to, such as textiles. The molecular targets include the hydroxyl and carboxyl groups, which participate in the formation of ester bonds and cross-links.
Comparison with Similar Compounds
Similar Compounds
Polyacrylic Acid: Another polymer used in textile finishing, but with different chemical properties and applications.
Polyvinyl Alcohol: Used in similar applications but differs in its chemical structure and properties.
Uniqueness
1,2,3,4-Butanetetracarboxylic acid polymer with 2,2-bis(hydroxymethyl)-1,3-propane-diol and 3-hydro is unique due to its specific combination of carboxyl and hydroxyl groups, which allow for versatile chemical modifications and applications. Its ability to form stable, cross-linked networks makes it particularly valuable in applications requiring enhanced durability and stability.
Properties
CAS No. |
101357-37-3 |
---|---|
Molecular Formula |
C27H49NO14 |
Molecular Weight |
611.7 g/mol |
IUPAC Name |
2,2-bis(hydroxymethyl)propane-1,3-diol;butane-1,2,3,4-tetracarboxylic acid;3-hydroxy-2,2-dimethyl-3-(2,2,6,6-tetramethylpiperidin-4-yl)propanal |
InChI |
InChI=1S/C14H27NO2.C8H10O8.C5H12O4/c1-12(2,9-16)11(17)10-7-13(3,4)15-14(5,6)8-10;9-5(10)1-3(7(13)14)4(8(15)16)2-6(11)12;6-1-5(2-7,3-8)4-9/h9-11,15,17H,7-8H2,1-6H3;3-4H,1-2H2,(H,9,10)(H,11,12)(H,13,14)(H,15,16);6-9H,1-4H2 |
InChI Key |
LMDRCXMICNBVHY-UHFFFAOYSA-N |
SMILES |
CC1(CC(CC(N1)(C)C)C(C(C)(C)C=O)O)C.C(C(C(CC(=O)O)C(=O)O)C(=O)O)C(=O)O.C(C(CO)(CO)CO)O |
Canonical SMILES |
CC1(CC(CC(N1)(C)C)C(C(C)(C)C=O)O)C.C(C(C(CC(=O)O)C(=O)O)C(=O)O)C(=O)O.C(C(CO)(CO)CO)O |
Synonyms |
1,2,3,4-Butanetetracarboxylic acid polymer with 2,2-bis(hydroxymethyl)-1,3-propane- diol and 3-hydroxy-2,2-dimethylpropanal, 2,2,6,6-tetramethyl-4-piperidinyl ester |
Origin of Product |
United States |
Disclaimer and Information on In-Vitro Research Products
Please be aware that all articles and product information presented on BenchChem are intended solely for informational purposes. The products available for purchase on BenchChem are specifically designed for in-vitro studies, which are conducted outside of living organisms. In-vitro studies, derived from the Latin term "in glass," involve experiments performed in controlled laboratory settings using cells or tissues. It is important to note that these products are not categorized as medicines or drugs, and they have not received approval from the FDA for the prevention, treatment, or cure of any medical condition, ailment, or disease. We must emphasize that any form of bodily introduction of these products into humans or animals is strictly prohibited by law. It is essential to adhere to these guidelines to ensure compliance with legal and ethical standards in research and experimentation.