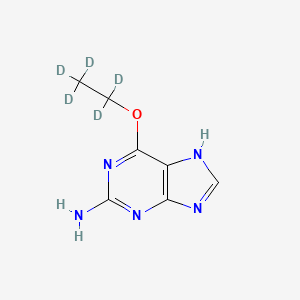
6-Ethyl Guanine-d5
- Click on QUICK INQUIRY to receive a quote from our team of experts.
- With the quality product at a COMPETITIVE price, you can focus more on your research.
Overview
Description
6-Ethyl Guanine-d5 is a deuterated compound, which means it contains deuterium, a stable isotope of hydrogen. This compound is a derivative of purine, a heterocyclic aromatic organic compound that plays a crucial role in biochemistry, particularly in the structure of DNA and RNA.
Mechanism of Action
Target of Action
6-Ethyl Guanine-d5, also known as 6-(1,1,2,2,2-Pentadeuterioethoxy)-7H-purin-2-amine, is a deuterated analogue of the purine nucleobase, Guanine Guanine-based purines, including guanosine, are known to interact with various targets such as g-proteins and p1 receptors .
Mode of Action
Guanine-based purines are known to play a crucial role in several intracellular processes, such as nucleic acid structure, energetic metabolism, and signal transduction via g-proteins .
Biochemical Pathways
Guanosine 5′-triphosphate (GTP) is a key substrate for the biosynthesis of guanosine 5′-diphosphate (GDP)-L-fucose . Guanosine nucleotides, including GMP, GDP, and GTP, are indispensable materials for cell growth and biosynthesis of DNA and RNA . They interconvert with each other through several reactions inside the cell .
Pharmacokinetics
The compound is soluble in water and common organic solvents like methanol, ethanol, and acetonitrile , which may influence its bioavailability.
Result of Action
Guanine-based purines have been shown to mediate several cellular processes, including cell growth, differentiation, and survival .
Action Environment
The compound is stable under normal conditions , suggesting that it may be resistant to various environmental factors.
Biochemical Analysis
Biochemical Properties
6-Ethyl Guanine-d5 is an alkylated mutagen of Guanine . The deuterium atoms replace the hydrogen atoms in the molecule, which makes it easier to distinguish from other compounds in the sample .
Cellular Effects
The cellular effects of this compound are not well-studied. Guanine, the base molecule of this compound, is known to be the most readily oxidized of the four DNA bases . This suggests that this compound may also be susceptible to oxidation, potentially influencing cellular processes such as DNA replication and repair .
Temporal Effects in Laboratory Settings
The temporal effects of this compound in laboratory settings are not well-documented. It is known that this compound is a stable compound, suggesting that it may have a long shelf-life and could be used in long-term studies .
Dosage Effects in Animal Models
The effects of this compound at different dosages in animal models are not well-studied. It is known that Guanine, the base molecule of this compound, is a crucial component of DNA and RNA, suggesting that changes in Guanine levels could potentially influence genetic processes .
Metabolic Pathways
This compound is likely involved in the purine metabolism pathway, given that Guanine, the base molecule of this compound, is a key component of this pathway . This pathway is crucial for the synthesis of DNA and RNA, and disruptions in this pathway can lead to various diseases .
Subcellular Localization
The subcellular localization of this compound is not well-studied. Given that Guanine, the base molecule of this compound, is a component of DNA and RNA, it is likely that this compound is localized to the nucleus and cytoplasm where these molecules are found .
Preparation Methods
Synthetic Routes and Reaction Conditions
The synthesis of 6-Ethyl Guanine-d5 typically involves the introduction of deuterium into the ethoxy group of the purine derivative. This can be achieved through various methods, including:
Deuterium Exchange Reactions: This involves the replacement of hydrogen atoms with deuterium in the presence of a deuterium source such as heavy water (D2O) or deuterated solvents.
Catalytic Hydrogenation: Using a deuterium gas (D2) in the presence of a catalyst to selectively hydrogenate the ethoxy group.
Industrial Production Methods
Industrial production of deuterated compounds often involves large-scale deuterium exchange reactions using deuterated solvents and catalysts to ensure high yield and purity. The process is optimized to minimize the loss of deuterium and to ensure the selective incorporation of deuterium into the desired positions.
Chemical Reactions Analysis
Types of Reactions
6-Ethyl Guanine-d5 can undergo various chemical reactions, including:
Oxidation: The compound can be oxidized to form corresponding oxides or other oxidation products.
Reduction: Reduction reactions can convert the compound into its reduced forms.
Substitution: The ethoxy group can be substituted with other functional groups under appropriate conditions.
Common Reagents and Conditions
Oxidation: Common oxidizing agents include potassium permanganate (KMnO4) and chromium trioxide (CrO3).
Reduction: Reducing agents such as lithium aluminum hydride (LiAlH4) or sodium borohydride (NaBH4) are commonly used.
Substitution: Nucleophilic substitution reactions can be carried out using reagents like sodium ethoxide (NaOEt) or potassium tert-butoxide (KOtBu).
Major Products
The major products formed from these reactions depend on the specific conditions and reagents used. For example, oxidation may yield purine oxides, while substitution reactions can produce various purine derivatives with different functional groups.
Scientific Research Applications
6-Ethyl Guanine-d5 has several scientific research applications:
Chemistry: Used as a stable isotope-labeled compound for studying reaction mechanisms and kinetics.
Biology: Employed in metabolic studies to trace biochemical pathways and understand the metabolism of purine derivatives.
Medicine: Investigated for its potential therapeutic applications, particularly in the development of deuterated drugs with improved pharmacokinetic properties.
Industry: Utilized in the production of deuterated materials for various industrial applications, including advanced materials and chemical synthesis.
Comparison with Similar Compounds
Similar Compounds
6-Ethoxy-7H-purin-2-amine: The non-deuterated analog of the compound.
6-(1,1,2,2,2-Pentadeuterioethoxy)-7H-purin-2-thiol: A sulfur analog with similar deuterium incorporation.
6-(1,1,2,2,2-Pentadeuterioethoxy)-7H-purin-2-ol: An alcohol analog with deuterium incorporation.
Uniqueness
The uniqueness of 6-Ethyl Guanine-d5 lies in its deuterium content, which provides enhanced stability and altered metabolic properties compared to its non-deuterated counterparts. This makes it valuable for research and potential therapeutic applications where improved stability and reduced metabolic degradation are desired.
Biological Activity
6-Ethyl Guanine-d5 is a deuterated derivative of guanine that has garnered attention in biochemical and pharmacological research due to its unique structural properties and biological activities. This compound is particularly significant in studies related to DNA alkylation, repair mechanisms, and potential therapeutic applications, including anticancer and antiviral activities.
Chemical Structure and Properties
This compound features an ethyl group at the 6-position of the guanine base, with deuterium isotopes replacing hydrogen atoms. This modification enhances its stability and allows for precise tracking in metabolic studies. The structural formula can be represented as follows:
1. Interaction with DNA
Research indicates that this compound can form adducts with DNA, particularly through alkylation processes. Alkylation of DNA can lead to mutations if not repaired, making the study of compounds like this compound crucial for understanding carcinogenesis. For instance, studies have shown that O6-ethylguanine (O6-EtGua), a product of alkylation by N-nitroso compounds, is associated with increased mutation rates in cells due to its mispairing during DNA replication .
2. Repair Mechanisms
The biological activity of this compound has also been explored in the context of DNA repair mechanisms. The presence of O6-alkylguanine-DNA alkyltransferase (AGT) is critical for repairing O6-EtGua lesions. Cells proficient in this repair mechanism exhibit lower rates of malignant transformation when exposed to alkylating agents compared to those deficient in AGT .
Case Studies and Experimental Findings
Several studies have investigated the effects of this compound on cellular systems:
- Study on Rat Fibroblasts : A study involving rat fibroblast cell lines demonstrated that variants expressing AGT were significantly less likely to develop tumors when exposed to alkylating agents compared to their AGT-deficient counterparts. The frequency of tumor formation was reduced by approximately 28-fold in AGT-positive cells .
- Persistence in DNA : Another investigation focused on the persistence of O6-EtGua in rat brain DNA, revealing that this compound remains detectable long after exposure to alkylating agents, indicating its potential role as a biomarker for DNA damage .
1. Anticancer Research
The ability of this compound to mimic natural guanine allows it to be utilized in cancer research, particularly in understanding how alterations in DNA structure can lead to malignancies. Its role in studying the efficacy of chemotherapeutic agents targeting alkylated DNA is particularly noteworthy.
2. Antiviral Potential
Emerging research suggests that compounds similar to this compound may exhibit antiviral properties by interfering with viral replication mechanisms. This aspect is under investigation, with preliminary data indicating potential pathways through which such compounds could inhibit viral activity.
Data Table: Summary of Biological Activities
Properties
IUPAC Name |
6-(1,1,2,2,2-pentadeuterioethoxy)-7H-purin-2-amine |
Source
|
---|---|---|
Source | PubChem | |
URL | https://pubchem.ncbi.nlm.nih.gov | |
Description | Data deposited in or computed by PubChem | |
InChI |
InChI=1S/C7H9N5O/c1-2-13-6-4-5(10-3-9-4)11-7(8)12-6/h3H,2H2,1H3,(H3,8,9,10,11,12)/i1D3,2D2 |
Source
|
Source | PubChem | |
URL | https://pubchem.ncbi.nlm.nih.gov | |
Description | Data deposited in or computed by PubChem | |
InChI Key |
SFXXRVSPZSOREJ-ZBJDZAJPSA-N |
Source
|
Source | PubChem | |
URL | https://pubchem.ncbi.nlm.nih.gov | |
Description | Data deposited in or computed by PubChem | |
Canonical SMILES |
CCOC1=NC(=NC2=C1NC=N2)N |
Source
|
Source | PubChem | |
URL | https://pubchem.ncbi.nlm.nih.gov | |
Description | Data deposited in or computed by PubChem | |
Isomeric SMILES |
[2H]C([2H])([2H])C([2H])([2H])OC1=NC(=NC2=C1NC=N2)N |
Source
|
Source | PubChem | |
URL | https://pubchem.ncbi.nlm.nih.gov | |
Description | Data deposited in or computed by PubChem | |
Molecular Formula |
C7H9N5O |
Source
|
Source | PubChem | |
URL | https://pubchem.ncbi.nlm.nih.gov | |
Description | Data deposited in or computed by PubChem | |
Molecular Weight |
184.21 g/mol |
Source
|
Source | PubChem | |
URL | https://pubchem.ncbi.nlm.nih.gov | |
Description | Data deposited in or computed by PubChem | |
Disclaimer and Information on In-Vitro Research Products
Please be aware that all articles and product information presented on BenchChem are intended solely for informational purposes. The products available for purchase on BenchChem are specifically designed for in-vitro studies, which are conducted outside of living organisms. In-vitro studies, derived from the Latin term "in glass," involve experiments performed in controlled laboratory settings using cells or tissues. It is important to note that these products are not categorized as medicines or drugs, and they have not received approval from the FDA for the prevention, treatment, or cure of any medical condition, ailment, or disease. We must emphasize that any form of bodily introduction of these products into humans or animals is strictly prohibited by law. It is essential to adhere to these guidelines to ensure compliance with legal and ethical standards in research and experimentation.