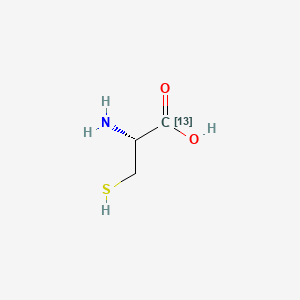
L-Cysteine-1-13C
Overview
Description
L-Cysteine-1-13C is a stable isotope-labeled compound of L-Cysteine, where the carbon-13 isotope is incorporated at the first carbon position. L-Cysteine is a conditionally essential amino acid that contains a thiol group, making it a crucial precursor for various biologically active molecules such as hydrogen sulfide, glutathione, and taurine. The incorporation of the carbon-13 isotope allows for detailed metabolic studies and tracing experiments in biochemical research.
Mechanism of Action
Target of Action
L-Cysteine-1-13C is a labeled variant of the amino acid L-Cysteine . The primary targets of L-Cysteine are the biological molecules that it acts as a precursor for, such as hydrogen sulphide (H2S), glutathione, and taurine .
Mode of Action
This compound interacts with its targets by providing the necessary sulfur atom for the synthesis of these molecules . The 13C label allows for the tracking of the compound’s metabolic pathway, providing valuable information about its interactions and transformations.
Biochemical Pathways
This compound is involved in several biochemical pathways due to its role as a precursor molecule. It is crucial in the synthesis of glutathione, a powerful antioxidant that protects cells from damage by reactive oxygen species . It also contributes to the production of taurine, an essential nutrient involved in various physiological processes .
Pharmacokinetics
The pharmacokinetics of this compound are similar to those of regular L-Cysteine. It is absorbed in the gastrointestinal tract and distributed throughout the body, including the brain .
Result of Action
The action of this compound results in the production of several biologically active molecules. For example, it contributes to the synthesis of glutathione, which plays a vital role in maintaining cellular redox balance and protecting cells from oxidative stress . It also suppresses ghrelin and reduces appetite in rodents and humans .
Action Environment
The action of this compound can be influenced by various environmental factors. For instance, the presence of other nutrients and the pH of the environment can affect its absorption and distribution . Additionally, certain pathological conditions, such as cancer, aging, diabetes, obesity, neurodegeneration, and chronic inflammatory diseases, can disrupt the balance between reductive and oxidative states, affecting the action of this compound .
Biochemical Analysis
Biochemical Properties
L-Cysteine-1-13C is involved in glutathione redox chemistry, which plays a central part of metabolic chemistry and strongly influences various therapies . It forms a disulfide bond in the presence of reduced glutathione, generating a spectroscopically detectable product .
Cellular Effects
This compound has a broad distribution throughout the body, including the brain . Its biochemical transformation in human pancreatic tumor cells in vitro and as xenografts differed depending on the individual cellular biochemical profile and microenvironment in vivo .
Molecular Mechanism
The molecular mechanism of this compound involves its interaction with glutathione. It forms a disulfide bond in the presence of reduced glutathione, which generates a spectroscopically detectable product .
Temporal Effects in Laboratory Settings
Its transformation in human pancreatic tumor cells was observed to differ depending on the individual cellular biochemical profile and microenvironment .
Metabolic Pathways
This compound is involved in glutathione redox chemistry, a crucial part of metabolic chemistry . It interacts with reduced glutathione, a key player in maintaining the oxidative balance in cells .
Transport and Distribution
This compound is broadly distributed throughout the body, including the brain
Preparation Methods
Synthetic Routes and Reaction Conditions
The synthesis of L-Cysteine-1-13C typically involves the incorporation of carbon-13 labeled precursors into the amino acid structure. One common method is the use of carbon-13 labeled acetic acid or other carbon-13 labeled compounds in the biosynthetic pathway of L-Cysteine. The reaction conditions often require controlled environments to ensure the incorporation of the isotope at the desired position.
Industrial Production Methods
Industrial production of this compound involves microbial fermentation using genetically engineered microorganisms. These microorganisms are designed to incorporate carbon-13 labeled substrates into their metabolic pathways, resulting in the production of this compound. The process includes fermentation, extraction, and purification steps to obtain the final product with high isotopic purity.
Chemical Reactions Analysis
Types of Reactions
L-Cysteine-1-13C undergoes various chemical reactions, including:
Oxidation: L-Cysteine can be oxidized to form cystine, a dimer linked by a disulfide bond.
Reduction: Cystine can be reduced back to L-Cysteine.
Substitution: The thiol group in L-Cysteine can participate in nucleophilic substitution reactions.
Common Reagents and Conditions
Oxidation: Hydrogen peroxide or other oxidizing agents are commonly used.
Reduction: Reducing agents such as dithiothreitol or β-mercaptoethanol are employed.
Substitution: Alkylating agents like iodoacetamide can react with the thiol group.
Major Products
Oxidation: Cystine
Reduction: L-Cysteine
Substitution: Alkylated cysteine derivatives
Scientific Research Applications
L-Cysteine-1-13C is widely used in scientific research due to its stable isotope labeling. Some applications include:
Metabolic Studies: Tracing metabolic pathways and studying the dynamics of sulfur-containing compounds.
Biological Research: Investigating the role of L-Cysteine in cellular processes and its involvement in redox reactions.
Medical Research: Exploring the therapeutic potential of L-Cysteine derivatives in treating diseases related to oxidative stress.
Industrial Applications: Used in the production of pharmaceuticals, food additives, and cosmetics.
Comparison with Similar Compounds
Similar Compounds
L-Cysteine: The non-labeled version of L-Cysteine.
L-Cystine: The oxidized dimer form of L-Cysteine.
N-Acetylcysteine: A derivative of L-Cysteine used as a medication and supplement.
Uniqueness
L-Cysteine-1-13C is unique due to the incorporation of the carbon-13 isotope, which allows for detailed metabolic tracing and studies. This makes it a valuable tool in research applications where understanding the metabolic fate of sulfur-containing compounds is essential.
Properties
IUPAC Name |
(2R)-2-amino-3-sulfanyl(113C)propanoic acid | |
---|---|---|
Source | PubChem | |
URL | https://pubchem.ncbi.nlm.nih.gov | |
Description | Data deposited in or computed by PubChem | |
InChI |
InChI=1S/C3H7NO2S/c4-2(1-7)3(5)6/h2,7H,1,4H2,(H,5,6)/t2-/m0/s1/i3+1 | |
Source | PubChem | |
URL | https://pubchem.ncbi.nlm.nih.gov | |
Description | Data deposited in or computed by PubChem | |
InChI Key |
XUJNEKJLAYXESH-NSQKCYGPSA-N | |
Source | PubChem | |
URL | https://pubchem.ncbi.nlm.nih.gov | |
Description | Data deposited in or computed by PubChem | |
Canonical SMILES |
C(C(C(=O)O)N)S | |
Source | PubChem | |
URL | https://pubchem.ncbi.nlm.nih.gov | |
Description | Data deposited in or computed by PubChem | |
Isomeric SMILES |
C([C@@H]([13C](=O)O)N)S | |
Source | PubChem | |
URL | https://pubchem.ncbi.nlm.nih.gov | |
Description | Data deposited in or computed by PubChem | |
Molecular Formula |
C3H7NO2S | |
Source | PubChem | |
URL | https://pubchem.ncbi.nlm.nih.gov | |
Description | Data deposited in or computed by PubChem | |
DSSTOX Substance ID |
DTXSID40745847 | |
Record name | L-(1-~13~C)Cysteine | |
Source | EPA DSSTox | |
URL | https://comptox.epa.gov/dashboard/DTXSID40745847 | |
Description | DSSTox provides a high quality public chemistry resource for supporting improved predictive toxicology. | |
Molecular Weight |
122.15 g/mol | |
Source | PubChem | |
URL | https://pubchem.ncbi.nlm.nih.gov | |
Description | Data deposited in or computed by PubChem | |
CAS No. |
224054-24-4 | |
Record name | L-(1-~13~C)Cysteine | |
Source | EPA DSSTox | |
URL | https://comptox.epa.gov/dashboard/DTXSID40745847 | |
Description | DSSTox provides a high quality public chemistry resource for supporting improved predictive toxicology. | |
Record name | 224054-24-4 | |
Source | European Chemicals Agency (ECHA) | |
URL | https://echa.europa.eu/information-on-chemicals | |
Description | The European Chemicals Agency (ECHA) is an agency of the European Union which is the driving force among regulatory authorities in implementing the EU's groundbreaking chemicals legislation for the benefit of human health and the environment as well as for innovation and competitiveness. | |
Explanation | Use of the information, documents and data from the ECHA website is subject to the terms and conditions of this Legal Notice, and subject to other binding limitations provided for under applicable law, the information, documents and data made available on the ECHA website may be reproduced, distributed and/or used, totally or in part, for non-commercial purposes provided that ECHA is acknowledged as the source: "Source: European Chemicals Agency, http://echa.europa.eu/". Such acknowledgement must be included in each copy of the material. ECHA permits and encourages organisations and individuals to create links to the ECHA website under the following cumulative conditions: Links can only be made to webpages that provide a link to the Legal Notice page. | |
Disclaimer and Information on In-Vitro Research Products
Please be aware that all articles and product information presented on BenchChem are intended solely for informational purposes. The products available for purchase on BenchChem are specifically designed for in-vitro studies, which are conducted outside of living organisms. In-vitro studies, derived from the Latin term "in glass," involve experiments performed in controlled laboratory settings using cells or tissues. It is important to note that these products are not categorized as medicines or drugs, and they have not received approval from the FDA for the prevention, treatment, or cure of any medical condition, ailment, or disease. We must emphasize that any form of bodily introduction of these products into humans or animals is strictly prohibited by law. It is essential to adhere to these guidelines to ensure compliance with legal and ethical standards in research and experimentation.