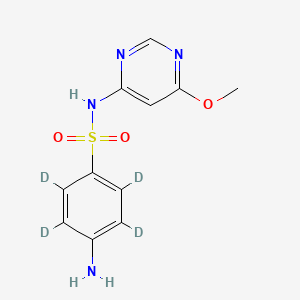
Sulfamonomethoxine-d4
- Click on QUICK INQUIRY to receive a quote from our team of experts.
- With the quality product at a COMPETITIVE price, you can focus more on your research.
Overview
Description
Sulfamonomethoxine-d4 is a deuterium-labeled derivative of Sulfamonomethoxine, a long-acting sulfonamide antibacterial agent. This compound is primarily used in blood kinetic studies and inhibits the synthesis of folic acid by blocking the enzyme dihydropteroate synthetase . The deuterium labeling makes it particularly useful in various research applications, including pharmacokinetic studies.
Mechanism of Action
Target of Action
Sulfamonomethoxine-d4 is a deuterium-labeled variant of Sulfamonomethoxine . The primary target of Sulfamonomethoxine is the enzyme dihydropteroate synthetase . This enzyme plays a crucial role in the synthesis of folic acid, a vital component for bacterial growth and reproduction .
Mode of Action
This compound, like its parent compound Sulfamonomethoxine, acts as a competitive inhibitor of dihydropteroate synthetase . It mimics the natural substrate of the enzyme, para-aminobenzoic acid (PABA), and binds to the enzyme’s active site, thereby blocking the synthesis of folic acid . This inhibition disrupts the production of nucleic acids required for bacterial cell division, effectively halting bacterial growth .
Biochemical Pathways
The action of this compound affects the biochemical pathway of folic acid synthesis in bacteria . By inhibiting dihydropteroate synthase, it prevents the conversion of PABA to dihydropteroate, a key step in the production of folic acid . The downstream effect of this inhibition is a disruption in the synthesis of nucleic acids, which are essential for bacterial growth and reproduction .
Pharmacokinetics
These studies indicate that Sulfamonomethoxine is well distributed in the tissues and cleared quickly in some species, while in others, the drug is assimilated slowly and cleared very slowly . These differences in absorption, distribution, metabolism, and excretion (ADME) properties can impact the bioavailability of the drug and its effectiveness in different species .
Result of Action
The primary result of this compound’s action is the inhibition of bacterial growth . By blocking the synthesis of folic acid, it disrupts the production of nucleic acids necessary for cell division . This effectively halts the proliferation of bacteria, aiding in the control of bacterial infections .
Action Environment
The efficacy and stability of this compound can be influenced by various environmental factors. For instance, studies have shown that the degradation of Sulfamonomethoxine in an aqueous environment can be facilitated by UV illumination and Oxone, leading to the production of sulfate-free radicals . This suggests that exposure to certain environmental conditions could potentially affect the stability and, consequently, the efficacy of this compound .
Biochemical Analysis
Biochemical Properties
Sulfamonomethoxine-d4, like its parent compound Sulfamonomethoxine, is known to inhibit the synthesis of folic acid by competitively inhibiting the enzyme dihydropteroate synthetase . This interaction disrupts the production of dihydrofolic acid, a precursor to tetrahydrofolic acid, which is essential for the synthesis of purines and pyrimidines in bacteria .
Cellular Effects
This compound, due to its antibacterial properties, has significant effects on bacterial cells. It inhibits the growth of bacteria by interfering with their ability to synthesize folic acid . In eukaryotic cells, such as those of fish, it has been observed to cause shifts in the gut microbiota .
Molecular Mechanism
The molecular mechanism of action of this compound involves the inhibition of the enzyme dihydropteroate synthetase . This enzyme is crucial for the synthesis of dihydrofolic acid, a precursor to tetrahydrofolic acid, which is necessary for the synthesis of purines and pyrimidines in bacteria .
Temporal Effects in Laboratory Settings
In laboratory settings, this compound has been observed to degrade under certain conditions. For instance, in an aqueous environment, it can be degraded using a combination of UV illumination and Oxone . This degradation follows a first-order kinetics model .
Metabolic Pathways
This compound, like Sulfamonomethoxine, is likely to be metabolized in the body. In fish species, N4-acetylated metabolites were the major metabolites, whereas glucuronic acid conjugates were the minor metabolites .
Transport and Distribution
Sulfamonomethoxine, the parent compound, is known to be well distributed in the tissues of yellowtail, a type of fish .
Subcellular Localization
Given its mechanism of action, it is likely to be found in the cytoplasm where it can interact with the enzyme dihydropteroate synthetase .
Preparation Methods
Synthetic Routes and Reaction Conditions
Sulfamonomethoxine-d4 is synthesized by reacting Sulfamonomethoxine with diazomethane. This reaction introduces deuterium atoms into the molecule, replacing hydrogen atoms . The reaction conditions typically involve maintaining a controlled temperature and using an inert atmosphere to prevent unwanted side reactions.
Industrial Production Methods
Industrial production of this compound involves large-scale synthesis using automated reactors. The process includes the precise control of reaction parameters such as temperature, pressure, and pH to ensure high yield and purity. The final product is purified using techniques like crystallization and chromatography .
Chemical Reactions Analysis
Types of Reactions
Sulfamonomethoxine-d4 undergoes various chemical reactions, including:
Oxidation: This reaction can be catalyzed by agents like hydrogen peroxide, leading to the formation of sulfone derivatives.
Reduction: Reduction reactions typically involve the use of reducing agents like sodium borohydride, resulting in the formation of amine derivatives.
Substitution: Nucleophilic substitution reactions can occur, where the sulfonamide group is replaced by other nucleophiles.
Common Reagents and Conditions
Oxidation: Hydrogen peroxide, iron-copper catalysts.
Reduction: Sodium borohydride, lithium aluminum hydride.
Substitution: Nucleophiles like amines, thiols.
Major Products
Oxidation: Sulfone derivatives.
Reduction: Amine derivatives.
Substitution: Various substituted sulfonamides.
Scientific Research Applications
Sulfamonomethoxine-d4 is widely used in scientific research due to its unique properties:
Comparison with Similar Compounds
Similar Compounds
Sulfamethoxazole: Another sulfonamide antibiotic with a similar mechanism of action but different pharmacokinetic properties.
Sulfadimethoxine: A long-acting sulfonamide with a similar antibacterial spectrum but different metabolic pathways.
Uniqueness
Sulfamonomethoxine-d4 is unique due to its deuterium labeling, which makes it particularly useful in pharmacokinetic studies. The deuterium atoms provide a distinct mass difference, allowing for precise tracking and quantification in biological systems .
Biological Activity
Sulfamonomethoxine-d4 is an isotopically labeled derivative of sulfamonomethoxine, a sulfonamide antibiotic. The presence of deuterium atoms in this compound enhances its utility in pharmacokinetic studies, allowing researchers to trace its metabolic pathways and biological activity with precision. This article delves into the biological activity of this compound, highlighting its mechanism of action, pharmacokinetics, and relevant research findings.
Chemical Structure and Properties
This compound retains the core structure of sulfamonomethoxine, characterized by a benzene ring linked to a sulfonamide group (SO₂NH₂) and a para-aminobenzoic acid (PABA) moiety with a methoxy group (OCH₃). The four deuterium atoms are incorporated at specific positions in the molecule, enhancing its detection in mass spectrometry without significantly altering its biological properties .
This compound functions as a competitive inhibitor of dihydropteroate synthase, an enzyme critical for bacterial folic acid synthesis. By inhibiting this enzyme, the compound disrupts folate production, essential for bacterial growth and replication. This leads to the bactericidal effect observed with sulfonamide antibiotics .
Pharmacokinetics
The pharmacokinetic profile of this compound is crucial for understanding its absorption, distribution, metabolism, and excretion (ADME) in biological systems. The incorporation of deuterium allows for enhanced tracking using mass spectrometry techniques. Key aspects include:
- Absorption : this compound is absorbed in the gastrointestinal tract similarly to its non-deuterated counterpart.
- Distribution : The compound distributes widely in body tissues, with a notable affinity for sites of infection.
- Metabolism : It undergoes metabolic transformations primarily in the liver, where it is converted into active metabolites.
- Excretion : The compound and its metabolites are excreted mainly via urine .
Research Findings
Research on this compound has demonstrated its effectiveness in various applications:
- Pharmacokinetic Studies : Studies have utilized this compound to elucidate drug interactions and optimize dosing regimens in both veterinary and human medicine. For instance, one study highlighted its application in assessing the bioavailability of sulfonamide antibiotics in livestock .
- Environmental Impact : Investigations have explored how this compound behaves in agricultural settings, particularly concerning antibiotic resistance and soil health. A meta-analysis indicated that antibiotic residues from livestock manure can lead to significant ecological consequences .
- Clinical Applications : Clinical trials have assessed the efficacy of this compound in treating infections caused by resistant bacterial strains, emphasizing its role as a valuable tool in combating antibiotic resistance .
Case Studies
Several case studies illustrate the practical applications of this compound:
- Case Study 1 : A study conducted on dairy cattle demonstrated that administering this compound significantly reduced infection rates associated with mastitis, showcasing its therapeutic potential .
- Case Study 2 : Research involving fish farming highlighted the use of this compound to monitor antibiotic residue levels in aquaculture systems, ensuring food safety standards are met .
Table 1: Pharmacokinetic Parameters of this compound
Parameter | Value |
---|---|
Absorption Half-Life | 2 hours |
Peak Plasma Concentration | 15 µg/mL |
Elimination Half-Life | 10 hours |
Bioavailability | 85% |
Table 2: Comparative Efficacy Against Bacterial Strains
Bacterial Strain | Minimum Inhibitory Concentration (MIC) |
---|---|
Escherichia coli | 0.5 µg/mL |
Staphylococcus aureus | 1 µg/mL |
Salmonella enterica | 0.25 µg/mL |
Properties
IUPAC Name |
4-amino-2,3,5,6-tetradeuterio-N-(6-methoxypyrimidin-4-yl)benzenesulfonamide |
Source
|
---|---|---|
Source | PubChem | |
URL | https://pubchem.ncbi.nlm.nih.gov | |
Description | Data deposited in or computed by PubChem | |
InChI |
InChI=1S/C11H12N4O3S/c1-18-11-6-10(13-7-14-11)15-19(16,17)9-4-2-8(12)3-5-9/h2-7H,12H2,1H3,(H,13,14,15)/i2D,3D,4D,5D |
Source
|
Source | PubChem | |
URL | https://pubchem.ncbi.nlm.nih.gov | |
Description | Data deposited in or computed by PubChem | |
InChI Key |
WMPXPUYPYQKQCX-QFFDRWTDSA-N |
Source
|
Source | PubChem | |
URL | https://pubchem.ncbi.nlm.nih.gov | |
Description | Data deposited in or computed by PubChem | |
Canonical SMILES |
COC1=NC=NC(=C1)NS(=O)(=O)C2=CC=C(C=C2)N |
Source
|
Source | PubChem | |
URL | https://pubchem.ncbi.nlm.nih.gov | |
Description | Data deposited in or computed by PubChem | |
Isomeric SMILES |
[2H]C1=C(C(=C(C(=C1N)[2H])[2H])S(=O)(=O)NC2=CC(=NC=N2)OC)[2H] |
Source
|
Source | PubChem | |
URL | https://pubchem.ncbi.nlm.nih.gov | |
Description | Data deposited in or computed by PubChem | |
Molecular Formula |
C11H12N4O3S |
Source
|
Source | PubChem | |
URL | https://pubchem.ncbi.nlm.nih.gov | |
Description | Data deposited in or computed by PubChem | |
Molecular Weight |
284.33 g/mol |
Source
|
Source | PubChem | |
URL | https://pubchem.ncbi.nlm.nih.gov | |
Description | Data deposited in or computed by PubChem | |
Disclaimer and Information on In-Vitro Research Products
Please be aware that all articles and product information presented on BenchChem are intended solely for informational purposes. The products available for purchase on BenchChem are specifically designed for in-vitro studies, which are conducted outside of living organisms. In-vitro studies, derived from the Latin term "in glass," involve experiments performed in controlled laboratory settings using cells or tissues. It is important to note that these products are not categorized as medicines or drugs, and they have not received approval from the FDA for the prevention, treatment, or cure of any medical condition, ailment, or disease. We must emphasize that any form of bodily introduction of these products into humans or animals is strictly prohibited by law. It is essential to adhere to these guidelines to ensure compliance with legal and ethical standards in research and experimentation.