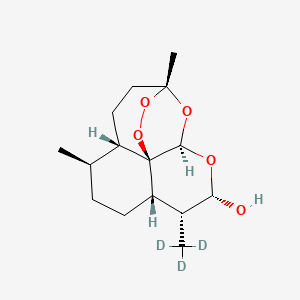
Dihydro Artemisinin-d3
- Click on QUICK INQUIRY to receive a quote from our team of experts.
- With the quality product at a COMPETITIVE price, you can focus more on your research.
Overview
Description
Dihydro Artemisinin-d3 is a deuterated derivative of dihydroartemisinin, which is a semi-synthetic derivative of artemisinin. Artemisinin is a natural compound extracted from the plant Artemisia annua, commonly known as sweet wormwood. This compound is primarily used in scientific research due to its enhanced stability and unique properties compared to its non-deuterated counterpart.
Mechanism of Action
Target of Action
Dihydro Artemisinin-d3 (DHA), a derivative of artemisinin, is primarily targeted against malaria parasites . It has been found to inhibit malignant tumor growth and regulate immune system function . The compound causes severe oxidative stress in parasites and tumors by inducing excessive reactive oxygen species production . It also enhances the ability of the immune system to resist parasites .
Mode of Action
DHA interacts with its targets by damaging their membranes, disrupting their mitochondrial function, and causing oxidative stress through producing excessive reactive oxide species (ROS) . It also kills tumor cells by inducing programmed cell death, blocking cell cycle, and enhancing anti-tumor immunity . In addition, DHA inhibits inflammation by reducing the inflammatory cells infiltration and suppressing the production of pro-inflammatory cytokines .
Biochemical Pathways
DHA affects several biochemical pathways. It regulates key factors such as apoptosis-related BAX, FASL and caspase-3, multi-drug resistance genes, cytokines such as CD4+ and CD8+, inflammation-related NF-κB and COX2, telomerase, and oxidative stress molecules . It also impacts the mevalonate pathway (MVA) and the cyclization of farnesyl diphosphate (FDP) .
Pharmacokinetics
DHA’s ADME properties impact its bioavailability. It is converted to DHA by esterases in the stomach . After intravenous injection, it has a steady-state volume of distribution of 0.39 L, and a clearance of 21–41 mL min^−1 kg^−1 . The terminal half-life after intravenous dosing is 1.35 h . Bioavailability after intramuscular injection is 85% . After oral administration, low bioavailability (19–35%) is observed .
Result of Action
The molecular and cellular effects of DHA’s action include the induction of T-cell activation, with an increased proportion of Ki67+CD4+ T cells, CD25+CD4+ T cells, interferon (IFN)-γ-producing CD8+ T cells, Brdu+ CD8+ T cells and neutrophils . This enhances cellular immunity to experimental malaria and overcomes immunosuppression in mice .
Action Environment
Environmental factors can influence DHA’s action, efficacy, and stability. For instance, the development of apparent artemisinin resistance in Cambodia is of particular concern . This phenomenon will become doubly alarming if resistance mechanisms evolve that affect a pathway common to both drugs within ACTs, through, for example, drug efflux mechanisms mediated by pfmdr1 copy number .
Biochemical Analysis
Biochemical Properties
Dihydro Artemisinin-d3 interacts with several enzymes, proteins, and other biomolecules. Experimental evidence suggests that this compound may exert its functions via mechanisms like regulating key factors such as apoptosis-related BAX, FASL and caspase-3, multi-drug resistance genes, cytokines such as CD4+ and CD8+, inflammation-related NF-κB and COX2, telomerase, and oxidative stress molecules .
Cellular Effects
This compound has been found to have significant effects on various types of cells and cellular processes. It causes severe oxidative stress in parasites and tumors by inducing excessive reactive oxygen species production . This compound also kills tumor cells by inducing programmed cell death, blocking the cell cycle, and enhancing anti-tumor immunity .
Molecular Mechanism
The molecular mechanism of this compound involves the cleavage of endoperoxide bridges by iron, producing free radicals which damage biological macromolecules causing oxidative stress in the cells of the parasite . It also inhibits the human acyltransferase, zinc finger DHHC domain-containing protein 6 (ZDHHC6), resulting in reduced palmitoylation and mislocalization of the small GTPase NRas in cancer cells .
Dosage Effects in Animal Models
In animal models, this compound has shown to improve cognitive functions in Alzheimer’s disease animal model 3xTg mice
Metabolic Pathways
This compound is involved in several metabolic pathways. Both artemether and artesunate are metabolised to this compound by rapid esteratic hydrolysis of artesunate or slower cytochrome P450 .
Transport and Distribution
This compound is transported into the cell and artemisinin-transferrin conjugate causes the release of iron which reductively cleaves the endoperoxide bridge
Subcellular Localization
This compound localizes to or near the endoplasmic reticulum and vesicles that mediate intracellular trafficking, including plasma membrane-associated cytostomes that import host hemoglobin to the digestive vacuole wherein artemisinin-activating heme moieties are released .
Preparation Methods
Synthetic Routes and Reaction Conditions
The synthesis of Dihydro Artemisinin-d3 involves the reduction of artemisinin using deuterated reducing agents. The process typically includes the following steps:
Reduction of Artemisinin: Artemisinin is reduced using deuterated borohydride to produce dihydroartemisinin-d3.
Purification: The product is purified using chromatographic techniques to ensure the removal of any impurities and unreacted starting materials.
Industrial Production Methods
Industrial production of this compound follows similar synthetic routes but on a larger scale. The process involves:
Large-scale Reduction: Utilizing industrial-grade deuterated reducing agents for the reduction of artemisinin.
Purification and Quality Control: Employing advanced purification techniques and stringent quality control measures to ensure the consistency and purity of the final product.
Chemical Reactions Analysis
Types of Reactions
Dihydro Artemisinin-d3 undergoes various chemical reactions, including:
Oxidation: It can be oxidized to form artemisinin derivatives.
Reduction: Further reduction can lead to the formation of more reduced artemisinin derivatives.
Substitution: It can undergo substitution reactions where hydrogen atoms are replaced by other functional groups.
Common Reagents and Conditions
Oxidation: Common oxidizing agents include hydrogen peroxide and peracids.
Reduction: Deuterated borohydride is commonly used for reduction.
Substitution: Various reagents such as halogens and alkylating agents are used for substitution reactions.
Major Products Formed
Oxidation Products: Various oxidized derivatives of artemisinin.
Reduction Products: More reduced forms of artemisinin derivatives.
Substitution Products: Substituted artemisinin derivatives with different functional groups.
Scientific Research Applications
Dihydro Artemisinin-d3 has a wide range of scientific research applications, including:
Chemistry: Used as a stable isotope-labeled compound in various chemical studies.
Biology: Employed in biological studies to understand the metabolism and mechanism of action of artemisinin derivatives.
Medicine: Investigated for its potential therapeutic effects in treating malaria and other diseases.
Industry: Used in the development of new pharmaceuticals and as a reference standard in analytical chemistry.
Comparison with Similar Compounds
Similar Compounds
Artemisinin: The parent compound from which dihydroartemisinin is derived.
Artemether: A methyl ether derivative of dihydroartemisinin.
Artesunate: A hemisuccinate derivative of dihydroartemisinin.
Arteether: An ethyl ether derivative of dihydroartemisinin.
Uniqueness of Dihydro Artemisinin-d3
This compound is unique due to its deuterated nature, which provides enhanced stability and allows for detailed metabolic studies. Its deuterium atoms replace hydrogen atoms, making it more resistant to metabolic degradation and providing a longer half-life in biological systems.
Properties
IUPAC Name |
(1R,4S,5R,8S,9R,10S,12R,13R)-1,5-dimethyl-9-(trideuteriomethyl)-11,14,15,16-tetraoxatetracyclo[10.3.1.04,13.08,13]hexadecan-10-ol |
Source
|
---|---|---|
Source | PubChem | |
URL | https://pubchem.ncbi.nlm.nih.gov | |
Description | Data deposited in or computed by PubChem | |
InChI |
InChI=1S/C15H24O5/c1-8-4-5-11-9(2)12(16)17-13-15(11)10(8)6-7-14(3,18-13)19-20-15/h8-13,16H,4-7H2,1-3H3/t8-,9-,10+,11+,12+,13-,14-,15-/m1/s1/i2D3 |
Source
|
Source | PubChem | |
URL | https://pubchem.ncbi.nlm.nih.gov | |
Description | Data deposited in or computed by PubChem | |
InChI Key |
BJDCWCLMFKKGEE-MPBQXAAISA-N |
Source
|
Source | PubChem | |
URL | https://pubchem.ncbi.nlm.nih.gov | |
Description | Data deposited in or computed by PubChem | |
Canonical SMILES |
CC1CCC2C(C(OC3C24C1CCC(O3)(OO4)C)O)C |
Source
|
Source | PubChem | |
URL | https://pubchem.ncbi.nlm.nih.gov | |
Description | Data deposited in or computed by PubChem | |
Isomeric SMILES |
[2H]C([2H])([2H])[C@@H]1[C@@H]2CC[C@H]([C@H]3[C@]24[C@H](O[C@@H]1O)O[C@@](CC3)(OO4)C)C |
Source
|
Source | PubChem | |
URL | https://pubchem.ncbi.nlm.nih.gov | |
Description | Data deposited in or computed by PubChem | |
Molecular Formula |
C15H24O5 |
Source
|
Source | PubChem | |
URL | https://pubchem.ncbi.nlm.nih.gov | |
Description | Data deposited in or computed by PubChem | |
Molecular Weight |
287.37 g/mol |
Source
|
Source | PubChem | |
URL | https://pubchem.ncbi.nlm.nih.gov | |
Description | Data deposited in or computed by PubChem | |
Disclaimer and Information on In-Vitro Research Products
Please be aware that all articles and product information presented on BenchChem are intended solely for informational purposes. The products available for purchase on BenchChem are specifically designed for in-vitro studies, which are conducted outside of living organisms. In-vitro studies, derived from the Latin term "in glass," involve experiments performed in controlled laboratory settings using cells or tissues. It is important to note that these products are not categorized as medicines or drugs, and they have not received approval from the FDA for the prevention, treatment, or cure of any medical condition, ailment, or disease. We must emphasize that any form of bodily introduction of these products into humans or animals is strictly prohibited by law. It is essential to adhere to these guidelines to ensure compliance with legal and ethical standards in research and experimentation.