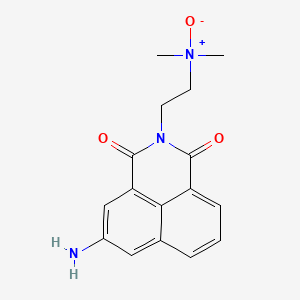
Amonafide N-Oxide
Overview
Description
Amonafide N-Oxide is a derivative of Amonafide, a compound that has been extensively studied for its potential in cancer treatment. Amonafide itself is known for its role as a DNA intercalating agent and topoisomerase II inhibitor, making it a promising candidate in oncology . The N-Oxide derivative retains many of these properties while potentially offering unique advantages in terms of reactivity and application.
Mechanism of Action
Target of Action
Amonafide N-Oxide primarily targets DNA topoisomerase 2-alpha and DNA topoisomerase 2-beta . These enzymes play a crucial role in DNA replication and transcription, and their inhibition can lead to cell death, particularly in rapidly dividing cells such as cancer cells .
Mode of Action
This compound acts as a DNA intercalating agent and an inhibitor of topoisomerase II . As an intercalating agent, it inserts itself between the DNA base pairs, causing distortions in the DNA helix that can inhibit DNA replication and transcription . As a topoisomerase II inhibitor, it prevents the re-ligation step of the DNA breakage-reunion process carried out by topoisomerase II, leading to DNA double-strand breaks .
Biochemical Pathways
Its mechanism of action suggests that it disrupts dna replication and transcription, which can affect numerous downstream cellular processes .
Pharmacokinetics
Studies on amonafide suggest that it is extensively metabolized and detected in plasma and urine . The initial plasma half-life of Amonafide is approximately 2.4 minutes, the intermediate half-life is around 26.8 minutes, and the terminal half-life is about 21.7 hours .
Result of Action
The primary result of this compound’s action is the induction of cell death in rapidly dividing cells, such as cancer cells . This is due to the disruption of DNA replication and transcription caused by its intercalation into DNA and inhibition of topoisomerase II .
Action Environment
The action, efficacy, and stability of this compound can be influenced by various environmental factors. For instance, the rate of N-acetylation can affect the drug’s toxicity, with fast acetylators experiencing greater myelosuppression than slow acetylators . Additionally, the presence of specific enzymes in the tumor microenvironment can potentially affect the activation and efficacy of the drug .
Biochemical Analysis
Biochemical Properties
Amonafide N-Oxide, like its parent compound Amonafide, is expected to interact with various enzymes and proteins. Amonafide has been shown to target topoisomerase II (Top2), stabilizing Top2 covalent complexes This interaction with Top2 is significant as it plays a crucial role in DNA replication and transcription
Cellular Effects
Amonafide has demonstrated significant activity in preclinical studies and some activity in Phase I trials . Its toxicity has been correlated to the formation of an active metabolite, N-acetyl-amonafide . It’s possible that this compound may have similar effects on cell function, including impacts on cell signaling pathways, gene expression, and cellular metabolism.
Molecular Mechanism
Amonafide is known to be a DNA intercalating agent and inhibitor of topoisomerase II . This suggests that this compound might exert its effects at the molecular level through similar mechanisms, including binding interactions with biomolecules, enzyme inhibition or activation, and changes in gene expression.
Temporal Effects in Laboratory Settings
Studies on Amonafide have shown that it undergoes extensive metabolism to at least three major metabolites and two or more minor metabolites .
Dosage Effects in Animal Models
The effects of this compound at different dosages in animal models are not yet fully known. Studies on Amonafide have shown that it has some activity in previously untreated breast cancer patients, with a significant correlation between myelosuppression and response .
Metabolic Pathways
The metabolic pathways that this compound is involved in are not yet fully known. Amonafide is known to be extensively metabolized and detected in plasma and urine . It’s plausible that this compound may be involved in similar metabolic pathways.
Transport and Distribution
Amonafide is known to penetrate the CSF readily and achieve the highest concentration 20–25 min after administration, which is 30% of the concurrent plasma level .
Subcellular Localization
The subcellular localization of this compound and any effects on its activity or function are not yet fully known. Amonafide is known to be a DNA intercalating agent , suggesting that it may localize to the nucleus where it interacts with DNA.
Preparation Methods
The synthesis of Amonafide N-Oxide typically involves the oxidation of Amonafide. Common oxidizing agents used for this purpose include hydrogen peroxide, sodium perborate, and urea-hydrogen peroxide adduct (UHP) . The reaction conditions often involve mild temperatures and the presence of catalysts such as rhenium-based compounds or titanium silicalite . Industrial production methods may employ continuous flow processes to enhance efficiency and yield .
Chemical Reactions Analysis
Amonafide N-Oxide undergoes various chemical reactions, including:
Oxidation: Further oxidation can lead to the formation of more highly oxidized species.
Reduction: Reduction reactions can convert the N-Oxide back to the parent amine or other reduced forms.
Substitution: N-Oxides can participate in nucleophilic substitution reactions, where the oxygen atom is replaced by other nucleophiles.
Common reagents for these reactions include reducing agents like hydrazine and sodium thiosulfate for reduction, and various nucleophiles for substitution reactions . Major products formed depend on the specific reaction conditions and reagents used.
Scientific Research Applications
Amonafide N-Oxide has a wide range of applications in scientific research:
Comparison with Similar Compounds
Amonafide N-Oxide can be compared to other topoisomerase inhibitors and DNA intercalators such as:
Mitoxantrone: Another topoisomerase II inhibitor but with a broader range of DNA cleavage sites.
Etoposide: A well-known topoisomerase II poison that also induces DNA strand breaks.
Naphthalimides: A class of compounds to which Amonafide belongs, known for their anticancer properties.
This compound is unique in its specific interaction with topoisomerase II and its potential for reduced side effects due to its selective action .
Properties
IUPAC Name |
2-(5-amino-1,3-dioxobenzo[de]isoquinolin-2-yl)-N,N-dimethylethanamine oxide | |
---|---|---|
Source | PubChem | |
URL | https://pubchem.ncbi.nlm.nih.gov | |
Description | Data deposited in or computed by PubChem | |
InChI |
InChI=1S/C16H17N3O3/c1-19(2,22)7-6-18-15(20)12-5-3-4-10-8-11(17)9-13(14(10)12)16(18)21/h3-5,8-9H,6-7,17H2,1-2H3 | |
Source | PubChem | |
URL | https://pubchem.ncbi.nlm.nih.gov | |
Description | Data deposited in or computed by PubChem | |
InChI Key |
LJUFWPUIUNYNJK-UHFFFAOYSA-N | |
Source | PubChem | |
URL | https://pubchem.ncbi.nlm.nih.gov | |
Description | Data deposited in or computed by PubChem | |
Canonical SMILES |
C[N+](C)(CCN1C(=O)C2=CC=CC3=CC(=CC(=C32)C1=O)N)[O-] | |
Source | PubChem | |
URL | https://pubchem.ncbi.nlm.nih.gov | |
Description | Data deposited in or computed by PubChem | |
Molecular Formula |
C16H17N3O3 | |
Source | PubChem | |
URL | https://pubchem.ncbi.nlm.nih.gov | |
Description | Data deposited in or computed by PubChem | |
DSSTOX Substance ID |
DTXSID80659055 | |
Record name | 2-(5-Amino-1,3-dioxo-1H-benzo[de]isoquinolin-2(3H)-yl)-N,N-dimethylethan-1-amine N-oxide | |
Source | EPA DSSTox | |
URL | https://comptox.epa.gov/dashboard/DTXSID80659055 | |
Description | DSSTox provides a high quality public chemistry resource for supporting improved predictive toxicology. | |
Molecular Weight |
299.32 g/mol | |
Source | PubChem | |
URL | https://pubchem.ncbi.nlm.nih.gov | |
Description | Data deposited in or computed by PubChem | |
CAS No. |
112726-97-3 | |
Record name | 1H-Benz(de)isoquinoline-1,3(2H)-dione, 5-amino-2-(2-(dimethylamino)ethyl)-, N-oxide | |
Source | ChemIDplus | |
URL | https://pubchem.ncbi.nlm.nih.gov/substance/?source=chemidplus&sourceid=0112726973 | |
Description | ChemIDplus is a free, web search system that provides access to the structure and nomenclature authority files used for the identification of chemical substances cited in National Library of Medicine (NLM) databases, including the TOXNET system. | |
Record name | 2-(5-Amino-1,3-dioxo-1H-benzo[de]isoquinolin-2(3H)-yl)-N,N-dimethylethan-1-amine N-oxide | |
Source | EPA DSSTox | |
URL | https://comptox.epa.gov/dashboard/DTXSID80659055 | |
Description | DSSTox provides a high quality public chemistry resource for supporting improved predictive toxicology. | |
Record name | 1H-BENZ(DE)ISOQUINOLINE-1,3(2H)-DIONE, 5-AMINO-2-(2-(DIMETHYLAMINO)ETHYL)-, N-OXIDE | |
Source | FDA Global Substance Registration System (GSRS) | |
URL | https://gsrs.ncats.nih.gov/ginas/app/beta/substances/BLW3994NJN | |
Description | The FDA Global Substance Registration System (GSRS) enables the efficient and accurate exchange of information on what substances are in regulated products. Instead of relying on names, which vary across regulatory domains, countries, and regions, the GSRS knowledge base makes it possible for substances to be defined by standardized, scientific descriptions. | |
Explanation | Unless otherwise noted, the contents of the FDA website (www.fda.gov), both text and graphics, are not copyrighted. They are in the public domain and may be republished, reprinted and otherwise used freely by anyone without the need to obtain permission from FDA. Credit to the U.S. Food and Drug Administration as the source is appreciated but not required. | |
Disclaimer and Information on In-Vitro Research Products
Please be aware that all articles and product information presented on BenchChem are intended solely for informational purposes. The products available for purchase on BenchChem are specifically designed for in-vitro studies, which are conducted outside of living organisms. In-vitro studies, derived from the Latin term "in glass," involve experiments performed in controlled laboratory settings using cells or tissues. It is important to note that these products are not categorized as medicines or drugs, and they have not received approval from the FDA for the prevention, treatment, or cure of any medical condition, ailment, or disease. We must emphasize that any form of bodily introduction of these products into humans or animals is strictly prohibited by law. It is essential to adhere to these guidelines to ensure compliance with legal and ethical standards in research and experimentation.