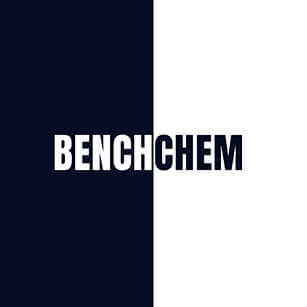
Allopurinol-d2
- Click on QUICK INQUIRY to receive a quote from our team of experts.
- With the quality product at a COMPETITIVE price, you can focus more on your research.
Overview
Description
Allopurinol-d2 is a deuterated form of allopurinol, a xanthine oxidase inhibitor primarily used to reduce uric acid levels in patients with gout and other conditions associated with hyperuricemia . The deuterium atoms in this compound replace the hydrogen atoms, which can be useful in various analytical and research applications due to the differences in mass and chemical properties.
Mechanism of Action
Target of Action
Allopurinol primarily targets the enzyme xanthine oxidase . This enzyme plays a crucial role in the conversion of hypoxanthine to xanthine and then to uric acid . By inhibiting xanthine oxidase, allopurinol prevents the formation of uric acid, which is the end product of purine metabolism .
Mode of Action
Allopurinol and its active metabolite, oxypurinol, inhibit the action of xanthine oxidase . They are also converted by the purine salvage pathway to their respective ribonucleotides . This interaction with the enzyme leads to a decrease in the production of uric acid without disrupting the biosynthesis of vital purines .
Biochemical Pathways
The inhibition of xanthine oxidase by allopurinol disrupts the biochemical pathway that leads to the formation of uric acid from hypoxanthine . This results in an increase in the levels of hypoxanthine and xanthine, which are more soluble and less likely to crystallize than uric acid . Furthermore, allopurinol can lead to the production of thioxanthine (2-hydroxymercaptopurine), which may inhibit thiopurine-S-methyltransferase (TPMT), providing a novel pathway of allopurinol-mediated TPMT inhibition .
Pharmacokinetics
Allopurinol has an oral bioavailability of approximately 79% and an elimination half-life of about 1.2 hours . It is rapidly and extensively metabolized to its active metabolite, oxypurinol . Oxypurinol has a longer half-life of around 23.3 hours and is cleared almost entirely by urinary excretion . The pharmacokinetics of allopurinol and oxypurinol are influenced by renal function, and dosage adjustments may be necessary in patients with renal impairment .
Result of Action
The primary result of allopurinol’s action is a reduction in the concentrations of uric acid in the blood and urine . This can help manage conditions like gout, where the deposition of uric acid crystals in body tissues leads to painful inflammation . By reducing uric acid production, allopurinol can decrease the frequency of gout attacks and prevent the formation of uric acid kidney stones .
Action Environment
The action of allopurinol can be influenced by various environmental factors. For instance, renal function significantly impacts the clearance of allopurinol and oxypurinol, affecting their concentrations and therapeutic effects . Additionally, the use of certain medications, such as uricosuric drugs, can affect the hypouricemic efficacy of allopurinol . These drugs increase the renal clearance of oxypurinol, potentially decreasing the influence of allopurinol . Therefore, the patient’s overall health status, concomitant medications, and other individual factors can influence the action, efficacy, and stability of allopurinol.
Biochemical Analysis
Biochemical Properties
Allopurinol-d2, like its parent compound allopurinol, plays a significant role in biochemical reactions. It inhibits the enzyme xanthine oxidase, which is involved in the conversion of hypoxanthine to xanthine and xanthine to uric acid . This interaction with xanthine oxidase is non-competitive and results in a decrease in the production of uric acid .
Cellular Effects
This compound impacts various types of cells and cellular processes. In human umbilical vein endothelial cells, allopurinol does not affect intracellular uric acid concentration . It also has an antioxidant property that might partially reverse endothelial dysfunction in patients with certain comorbidities .
Molecular Mechanism
The molecular mechanism of action of this compound is primarily through its inhibition of the enzyme xanthine oxidase. This inhibition blocks the biochemical reactions that lead to the formation of uric acid . Allopurinol also has theoretical interest. In 1996, Begoña Hernández, Francisco J. Luque, and Modesto Orozco at the University of Barcelona used computational methods to evaluate the relative stabilities of tautomers of allopurinol and its isomer hypoxanthine .
Temporal Effects in Laboratory Settings
The effects of this compound over time in laboratory settings have been observed in several studies. Allopurinol and its derivative have been shown to induce significant reduction in body weight, systolic blood pressure, blood glucose, insulin, lipids, and improved kidney functions and endothelial integrity compared to non-treated rats .
Dosage Effects in Animal Models
In animal models, the effects of this compound vary with different dosages. For instance, in a study comparing the effects of febuxostat and allopurinol in a rat model of metabolic syndrome, it was found that febuxostat was more effective than allopurinol in normalizing serum fasting glucose, uric acid, catalase, and glutathione peroxidase activities .
Metabolic Pathways
This compound is involved in purine metabolism, specifically in the catabolism of hypoxanthine and xanthine to uric acid. It inhibits the enzyme xanthine oxidase, thereby blocking this metabolic pathway . This leads to a decrease in the production of uric acid and an increase in the levels of hypoxanthine and xanthine .
Transport and Distribution
The transport and distribution of this compound within cells and tissues are influenced by its interaction with various transporters. For example, in human umbilical vein endothelial cells, allopurinol does not affect intracellular uric acid concentration .
Preparation Methods
Synthetic Routes and Reaction Conditions
The synthesis of allopurinol-d2 involves the incorporation of deuterium into the allopurinol molecule. One common method is the exchange of hydrogen atoms with deuterium using deuterated reagents under specific conditions. For example, allopurinol can be reacted with deuterated water (D2O) in the presence of a catalyst to achieve the desired deuteration .
Industrial Production Methods
Industrial production of this compound typically involves large-scale deuteration processes. These processes are optimized for high yield and purity, often using advanced techniques such as continuous flow reactors and specialized catalysts to facilitate the exchange of hydrogen with deuterium .
Chemical Reactions Analysis
Types of Reactions
Allopurinol-d2, like its non-deuterated counterpart, undergoes various chemical reactions, including:
Oxidation: this compound can be oxidized to form oxypurinol-d2, which retains the deuterium atoms.
Reduction: Reduction reactions can be used to modify the functional groups in this compound.
Substitution: Deuterium atoms in this compound can be replaced with other substituents under specific conditions.
Common Reagents and Conditions
Common reagents used in the reactions of this compound include deuterated solvents, catalysts, and oxidizing or reducing agents. Reaction conditions such as temperature, pressure, and pH are carefully controlled to achieve the desired outcomes .
Major Products Formed
The major products formed from the reactions of this compound include oxypurinol-d2 and other deuterated derivatives, which are useful in various research and analytical applications .
Scientific Research Applications
Allopurinol-d2 has a wide range of scientific research applications, including:
Comparison with Similar Compounds
Similar Compounds
Allopurinol: The non-deuterated form of allopurinol-d2, widely used in the treatment of gout and hyperuricemia.
Febuxostat: Another xanthine oxidase inhibitor used as an alternative to allopurinol.
Oxypurinol: The active metabolite of allopurinol, which also inhibits xanthine oxidase.
Uniqueness
This compound is unique due to the presence of deuterium atoms, which provide distinct advantages in research applications. The differences in mass and chemical properties between deuterium and hydrogen can be exploited in various analytical techniques, making this compound a valuable tool in scientific studies .
Properties
CAS No. |
916979-34-5 |
---|---|
Molecular Formula |
C5H4N4O |
Molecular Weight |
138.12 g/mol |
IUPAC Name |
3,6-dideuterio-1,2-dihydropyrazolo[3,4-d]pyrimidin-4-one |
InChI |
InChI=1S/C5H4N4O/c10-5-3-1-8-9-4(3)6-2-7-5/h1-2H,(H2,6,7,8,9,10)/i1D,2D |
InChI Key |
OFCNXPDARWKPPY-QDNHWIQGSA-N |
Isomeric SMILES |
[2H]C1=C2C(=NC(=NC2=O)[2H])NN1 |
SMILES |
C1=C2C(=NC=NC2=O)NN1 |
Canonical SMILES |
C1=C2C(=NC=NC2=O)NN1 |
Synonyms |
1,5-Dihydro-4H-pyrazolo[3,4-d]pyrimidin-4-one-d2; 4-Hydroxypyrazolo[3,4-d]pyrimidine-d2; 4-Oxopyrazolo[3,4-d]pyrimidine-d2; Adenock-d2; Allopur-d2; Caplenal-d2; Cellidrin-d2; NSC 101655-d2; NSC 1390-d2; |
Origin of Product |
United States |
Retrosynthesis Analysis
AI-Powered Synthesis Planning: Our tool employs the Template_relevance Pistachio, Template_relevance Bkms_metabolic, Template_relevance Pistachio_ringbreaker, Template_relevance Reaxys, Template_relevance Reaxys_biocatalysis model, leveraging a vast database of chemical reactions to predict feasible synthetic routes.
One-Step Synthesis Focus: Specifically designed for one-step synthesis, it provides concise and direct routes for your target compounds, streamlining the synthesis process.
Accurate Predictions: Utilizing the extensive PISTACHIO, BKMS_METABOLIC, PISTACHIO_RINGBREAKER, REAXYS, REAXYS_BIOCATALYSIS database, our tool offers high-accuracy predictions, reflecting the latest in chemical research and data.
Strategy Settings
Precursor scoring | Relevance Heuristic |
---|---|
Min. plausibility | 0.01 |
Model | Template_relevance |
Template Set | Pistachio/Bkms_metabolic/Pistachio_ringbreaker/Reaxys/Reaxys_biocatalysis |
Top-N result to add to graph | 6 |
Feasible Synthetic Routes
Disclaimer and Information on In-Vitro Research Products
Please be aware that all articles and product information presented on BenchChem are intended solely for informational purposes. The products available for purchase on BenchChem are specifically designed for in-vitro studies, which are conducted outside of living organisms. In-vitro studies, derived from the Latin term "in glass," involve experiments performed in controlled laboratory settings using cells or tissues. It is important to note that these products are not categorized as medicines or drugs, and they have not received approval from the FDA for the prevention, treatment, or cure of any medical condition, ailment, or disease. We must emphasize that any form of bodily introduction of these products into humans or animals is strictly prohibited by law. It is essential to adhere to these guidelines to ensure compliance with legal and ethical standards in research and experimentation.