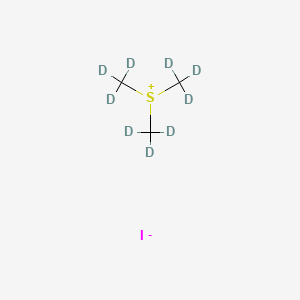
Trimethyl-D9-sulfonium iodide
- Click on QUICK INQUIRY to receive a quote from our team of experts.
- With the quality product at a COMPETITIVE price, you can focus more on your research.
Overview
Description
Trimethyl-D9-sulfonium iodide is a stable isotope-labeled compound, often used in various scientific research applications. It is a derivative of trimethylsulfonium iodide, where the hydrogen atoms are replaced with deuterium (D9), making it useful in nuclear magnetic resonance (NMR) studies and other analytical techniques .
Mechanism of Action
Target of Action
Trimethyl-D9-sulfonium iodide primarily targets carbonyl compounds , specifically ketones and aldehydes . These compounds play a crucial role in various biochemical reactions, serving as intermediates in metabolic pathways.
Mode of Action
This compound interacts with its targets through a process known as the Corey-Chaykovsky Reaction . This reaction begins with the deprotonation of sulfonium halides with strong bases to generate ylides . The ylide then acts as a nucleophile, attacking the carbonyl compound . The resulting oxygen anion reacts as an intramolecular nucleophile towards the electrophilic ylide carbon, which bears a sulfonium cation as a good leaving group .
Biochemical Pathways
The interaction of this compound with carbonyl compounds leads to the formation of epoxides . Epoxides are cyclic ethers that are involved in various biochemical pathways. They can undergo reactions to form a variety of other compounds, serving as versatile intermediates in organic synthesis .
Result of Action
The primary result of this compound’s action is the formation of epoxides from carbonyl compounds . Epoxides are valuable in organic synthesis due to their ability to undergo a variety of reactions, leading to the formation of a wide range of products.
Action Environment
The action of this compound can be influenced by various environmental factors. For instance, the presence of a strong base is necessary for the generation of the ylide that initiates the Corey-Chaykovsky Reaction . Additionally, the reaction’s efficiency can be affected by the solvent used, the temperature, and the presence of other substances that can react with the ylide or the carbonyl compound .
Biochemical Analysis
Biochemical Properties
Trimethyl-D9-sulfonium iodide is known to play a role in biochemical reactions. It is used to generate dimethyloxosulfonium methylide by reaction with sodium hydride . This compound is used as a methylene-transfer reagent, and is used to prepare epoxides
Molecular Mechanism
This compound exerts its effects at the molecular level through its conversion to dimethyloxosulfonium methylide . This compound can react with the carbonyl group of ketones to form epoxides
Preparation Methods
Synthetic Routes and Reaction Conditions: Trimethyl-D9-sulfonium iodide can be synthesized by reacting trimethylsulfonium iodide with deuterated methyl iodide (CD3I). The reaction typically occurs in an inert atmosphere to prevent any unwanted side reactions. The reaction is as follows:
(CD3)2S+CD3I→[(CD3)3S]+I−
Industrial Production Methods: In industrial settings, the production of this compound involves large-scale reactions under controlled conditions. The use of deuterated reagents ensures the incorporation of deuterium atoms, which is crucial for its application in NMR studies .
Chemical Reactions Analysis
Types of Reactions: Trimethyl-D9-sulfonium iodide undergoes several types of chemical reactions, including:
Substitution Reactions: It can participate in nucleophilic substitution reactions where the iodide ion is replaced by other nucleophiles.
Oxidation Reactions: It can be oxidized to form sulfoxides or sulfones under appropriate conditions.
Common Reagents and Conditions:
Nucleophiles: Such as hydroxide ions (OH^-), cyanide ions (CN^-), and thiolate ions (RS^-).
Oxidizing Agents: Such as hydrogen peroxide (H2O2) and potassium permanganate (KMnO4).
Major Products:
Sulfoxides and Sulfones: Formed through oxidation reactions.
Substituted Sulfonium Compounds: Formed through nucleophilic substitution reactions.
Scientific Research Applications
Trimethyl-D9-sulfonium iodide is widely used in scientific research due to its unique properties:
NMR Studies: The deuterium atoms make it an excellent internal standard for NMR spectroscopy, providing accurate and reliable data.
Analytical Chemistry: Used as a reference material in mass spectrometry and other analytical techniques.
Biological Studies: Helps in tracing metabolic pathways and studying enzyme mechanisms.
Industrial Applications: Used in the synthesis of deuterated compounds for various industrial applications
Comparison with Similar Compounds
- Trimethylsulfonium iodide
- Trimethylsulfoxonium iodide
- Dimethylsulfonium methylide
Uniqueness: Trimethyl-D9-sulfonium iodide is unique due to the presence of deuterium atoms, which enhance its utility in NMR studies and other analytical applications. The deuterium labeling provides distinct advantages in tracing and studying complex chemical and biological processes .
Biological Activity
Trimethyl-D9-sulfonium iodide (CAS Number: 106776-17-4) is a stable isotope-labeled compound derived from trimethylsulfonium iodide, where hydrogen atoms are replaced with deuterium. This unique labeling enhances its utility in various scientific applications, particularly in nuclear magnetic resonance (NMR) spectroscopy and analytical chemistry. Understanding its biological activity is crucial for its application in biochemical research and industrial processes.
This compound primarily interacts with carbonyl compounds, specifically ketones and aldehydes, through a reaction mechanism known as the Corey-Chaykovsky reaction. This process leads to the formation of epoxides from carbonyl substrates, which are significant intermediates in organic synthesis.
Corey-Chaykovsky Reaction
The Corey-Chaykovsky reaction involves the generation of a ylide from this compound in the presence of a strong base. The ylide subsequently reacts with carbonyl compounds to form epoxides, which can be further transformed into various functional groups.
This compound serves as a precursor for generating dimethyloxosulfonium methylide when reacted with sodium hydride. This conversion is essential for studying metabolic pathways and enzyme mechanisms, making it a valuable tool in biochemical research.
Chemical Reactions
The compound undergoes several types of chemical reactions:
- Nucleophilic Substitution Reactions : The iodide ion can be replaced by various nucleophiles.
- Oxidation Reactions : Under specific conditions, it can be oxidized to form sulfoxides or sulfones.
Reaction Type | Description | Major Products |
---|---|---|
Nucleophilic Substitution | Replacement of iodide by nucleophiles | Substituted sulfonium compounds |
Oxidation | Conversion to sulfoxides or sulfones | Sulfoxides, sulfones |
Applications in Scientific Research
This compound is widely utilized in various fields due to its unique properties:
- NMR Studies : The presence of deuterium provides an excellent internal standard for NMR spectroscopy, facilitating accurate data collection.
- Analytical Chemistry : It acts as a reference material in mass spectrometry and other analytical techniques.
- Biological Studies : The compound aids in tracing metabolic pathways and studying enzyme mechanisms, particularly in drug metabolism and pharmacokinetics.
- Industrial Applications : It is used in synthesizing deuterated compounds for pharmaceutical and agrochemical industries.
Case Studies
- NMR Spectroscopy : In studies involving metabolic tracing, this compound has been used to analyze the metabolism of carbonyl compounds in biological systems. Its deuterated form allows researchers to monitor reactions without interfering with the natural abundance of hydrogen isotopes.
- Enzyme Mechanism Studies : Research has demonstrated that this compound can effectively inhibit certain enzymes involved in metabolic processes. For instance, studies have shown that it can influence the activity of acetylcholinesterase (AChE), an enzyme critical for neurotransmission.
Properties
IUPAC Name |
tris(trideuteriomethyl)sulfanium;iodide |
Source
|
---|---|---|
Source | PubChem | |
URL | https://pubchem.ncbi.nlm.nih.gov | |
Description | Data deposited in or computed by PubChem | |
InChI |
InChI=1S/C3H9S.HI/c1-4(2)3;/h1-3H3;1H/q+1;/p-1/i1D3,2D3,3D3; |
Source
|
Source | PubChem | |
URL | https://pubchem.ncbi.nlm.nih.gov | |
Description | Data deposited in or computed by PubChem | |
InChI Key |
VFJYIHQDILEQNR-KYRNGWDOSA-M |
Source
|
Source | PubChem | |
URL | https://pubchem.ncbi.nlm.nih.gov | |
Description | Data deposited in or computed by PubChem | |
Canonical SMILES |
C[S+](C)C.[I-] |
Source
|
Source | PubChem | |
URL | https://pubchem.ncbi.nlm.nih.gov | |
Description | Data deposited in or computed by PubChem | |
Isomeric SMILES |
[2H]C([2H])([2H])[S+](C([2H])([2H])[2H])C([2H])([2H])[2H].[I-] |
Source
|
Source | PubChem | |
URL | https://pubchem.ncbi.nlm.nih.gov | |
Description | Data deposited in or computed by PubChem | |
Molecular Formula |
C3H9IS |
Source
|
Source | PubChem | |
URL | https://pubchem.ncbi.nlm.nih.gov | |
Description | Data deposited in or computed by PubChem | |
Molecular Weight |
213.13 g/mol |
Source
|
Source | PubChem | |
URL | https://pubchem.ncbi.nlm.nih.gov | |
Description | Data deposited in or computed by PubChem | |
Disclaimer and Information on In-Vitro Research Products
Please be aware that all articles and product information presented on BenchChem are intended solely for informational purposes. The products available for purchase on BenchChem are specifically designed for in-vitro studies, which are conducted outside of living organisms. In-vitro studies, derived from the Latin term "in glass," involve experiments performed in controlled laboratory settings using cells or tissues. It is important to note that these products are not categorized as medicines or drugs, and they have not received approval from the FDA for the prevention, treatment, or cure of any medical condition, ailment, or disease. We must emphasize that any form of bodily introduction of these products into humans or animals is strictly prohibited by law. It is essential to adhere to these guidelines to ensure compliance with legal and ethical standards in research and experimentation.