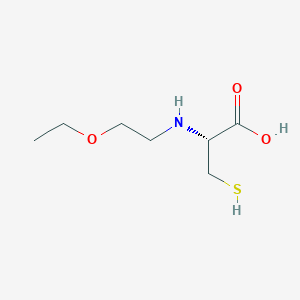
2-Ethoxyethyl-L-cysteine
Overview
Description
2-Ethoxyethyl-L-cysteine is a derivative of the amino acid L-cysteine. It is characterized by the presence of an ethoxyethyl group attached to the sulfur atom of the cysteine molecule.
Mechanism of Action
Target of Action
It is known that l-cysteine, a related compound, plays a crucial role in the cysteine biosynthetic pathway . The enzyme CysE, a Serine Acetyltransferase (SAT), catalyzes the first step of cysteine synthesis and is reported to be essential for the survival of persistence in several microbes
Mode of Action
It is known that l-cysteine and its derivatives can act as antioxidants and glutathione inducers . They can also be used as mucolytic agents in patients with certain lung conditions . It is possible that 2-Ethoxyethyl-L-cysteine may have similar interactions with its targets, leading to changes in cellular processes.
Biochemical Pathways
For instance, it is a key component in the cysteine biosynthetic pathway . It is also involved in the production of glutathione, a crucial antioxidant in the body . It is plausible that this compound may affect similar pathways, but more research is needed to confirm this.
Pharmacokinetics
After oral administration, N-acetylcysteine is rapidly absorbed, reaching peak plasma concentrations within 1 to 2 hours . The half-life is approximately 15-18 hours
Result of Action
For instance, they can act as antioxidants, neutralizing harmful free radicals in the body . They can also induce the production of glutathione, a crucial antioxidant in the body . It is plausible that this compound may have similar effects, but more research is needed to confirm this.
Biochemical Analysis
Biochemical Properties
It is known that cysteine, a related compound, plays a crucial role in protein folding, assembly, and stability through disulfide-bond formation . It is also involved in protecting cells under oxidative stress in vivo .
Cellular Effects
It is known that cysteine, a related compound, plays a crucial role in cellular metabolism .
Molecular Mechanism
It is known that cysteine, a related compound, is involved in the biosynthesis of sulfur-containing compounds such as L-methionine, thiamine, biotin, coenzyme A, and others .
Metabolic Pathways
It is known that cysteine, a related compound, is involved in the biosynthesis of sulfur-containing compounds such as L-methionine, thiamine, biotin, coenzyme A, and others .
Preparation Methods
Synthetic Routes and Reaction Conditions
The synthesis of 2-Ethoxyethyl-L-cysteine typically involves the reaction of L-cysteine with 2-ethoxyethanol in the presence of a suitable catalyst. The reaction is carried out under controlled conditions to ensure the selective formation of the desired product. The general reaction scheme can be represented as follows:
L-cysteine+2-ethoxyethanol→this compound
The reaction conditions, such as temperature, pH, and reaction time, are optimized to achieve high yield and purity of the product .
Industrial Production Methods
In an industrial setting, the production of this compound may involve large-scale batch or continuous processes. The use of advanced reactors and purification techniques ensures the efficient production of the compound with minimal impurities. The industrial process may also incorporate steps for the recovery and recycling of solvents and catalysts to enhance sustainability .
Chemical Reactions Analysis
Types of Reactions
2-Ethoxyethyl-L-cysteine can undergo various chemical reactions, including:
Oxidation: The sulfur atom in the cysteine moiety can be oxidized to form sulfoxides or sulfones.
Reduction: The compound can be reduced to yield the corresponding thiol derivative.
Substitution: The ethoxyethyl group can be substituted with other functional groups under appropriate conditions.
Common Reagents and Conditions
Oxidation: Common oxidizing agents such as hydrogen peroxide or potassium permanganate can be used.
Reduction: Reducing agents like sodium borohydride or lithium aluminum hydride are typically employed.
Substitution: Nucleophilic substitution reactions can be carried out using reagents like alkyl halides or acyl chlorides.
Major Products Formed
Oxidation: Sulfoxides and sulfones.
Reduction: Thiol derivatives.
Substitution: Various substituted cysteine derivatives.
Scientific Research Applications
2-Ethoxyethyl-L-cysteine has a wide range of applications in scientific research:
Chemistry: It is used as a building block for the synthesis of more complex molecules and as a reagent in various chemical reactions.
Biology: The compound is studied for its potential role in modulating biological processes, including enzyme activity and protein function.
Medicine: Research is ongoing to explore its therapeutic potential in treating diseases related to oxidative stress and inflammation.
Industry: It is used in the development of novel materials and as an additive in various industrial processes.
Comparison with Similar Compounds
Similar Compounds
L-cysteine: The parent amino acid, which lacks the ethoxyethyl group.
N-acetyl-L-cysteine: A derivative of L-cysteine with an acetyl group attached to the amino group.
S-methyl-L-cysteine: A derivative with a methyl group attached to the sulfur atom.
Uniqueness
2-Ethoxyethyl-L-cysteine is unique due to the presence of the ethoxyethyl group, which imparts distinct chemical and biological properties. This modification can enhance the compound’s solubility, stability, and reactivity compared to its parent compound and other derivatives .
Biological Activity
2-Ethoxyethyl-L-cysteine (EE-L-Cys) is a derivative of L-cysteine, an amino acid known for its biological significance, particularly in antioxidant defense and cellular metabolism. This article explores the biological activity of EE-L-Cys, focusing on its mechanisms of action, pharmacokinetics, and potential therapeutic applications.
Antioxidant Properties
EE-L-Cys is believed to exhibit antioxidant properties similar to those of its parent compound, L-cysteine. It can act as a precursor to glutathione, a critical antioxidant in the body that helps neutralize reactive oxygen species (ROS) and protects cells from oxidative stress. This property is crucial for maintaining cellular health and preventing damage associated with various diseases, including cancer and neurodegenerative disorders .
Modulation of Enzyme Activity
Research indicates that EE-L-Cys may modulate enzyme activities involved in metabolic pathways. It has been shown to influence the cysteine biosynthetic pathway, which is vital for synthesizing proteins and other biomolecules essential for cellular function. Additionally, it may affect the activity of enzymes that utilize cysteine as a substrate, thereby impacting various biochemical processes.
Pharmacokinetics
The pharmacokinetic profile of EE-L-Cys remains largely under-researched; however, insights can be drawn from studies on L-cysteine. After oral administration, L-cysteine is rapidly absorbed, reaching peak plasma concentrations within 1 to 2 hours. Similar absorption characteristics are anticipated for EE-L-Cys, although specific studies are needed to confirm this.
Table 1: Summary of Toxicity Studies on L-Cysteine
Dose (mg/kg/day) | Observed Effects | Control Group Effects |
---|---|---|
500 | Mild renal changes | No significant changes |
1000 | Moderate renal injuries | No significant changes |
2000 | Severe renal injuries; mortality risk | No significant changes |
These findings suggest that while L-cysteine can exhibit toxicity at high doses, further research is needed to establish a comprehensive safety profile for EE-L-Cys.
Potential Therapeutic Applications
Neuroprotection
Given its antioxidant properties, EE-L-Cys may have potential applications in neuroprotection. Studies have indicated that compounds enhancing glutathione levels can protect against neurodegenerative diseases such as Alzheimer's and Parkinson's disease . The ability of EE-L-Cys to scavenge ROS could be beneficial in mitigating oxidative damage in neural tissues.
Cancer Therapy
EE-L-Cys might also play a role in cancer therapy by modulating redox status within tumor cells. By influencing the balance between oxidants and antioxidants, it could potentially enhance the efficacy of chemotherapeutic agents while protecting normal cells from oxidative damage .
Properties
CAS No. |
885457-17-0 |
---|---|
Molecular Formula |
C7H15NO3S |
Molecular Weight |
193.27 g/mol |
IUPAC Name |
(2R)-2-amino-3-(2-ethoxyethylsulfanyl)propanoic acid |
InChI |
InChI=1S/C7H15NO3S/c1-2-11-3-4-12-5-6(8)7(9)10/h6H,2-5,8H2,1H3,(H,9,10)/t6-/m0/s1 |
InChI Key |
ALMNPOWSXDDYKJ-LURJTMIESA-N |
SMILES |
CCOCCNC(CS)C(=O)O |
Isomeric SMILES |
CCOCCSC[C@@H](C(=O)O)N |
Canonical SMILES |
CCOCCSCC(C(=O)O)N |
Synonyms |
3-(2-Ethoxyethylthio)alanine; |
Origin of Product |
United States |
Retrosynthesis Analysis
AI-Powered Synthesis Planning: Our tool employs the Template_relevance Pistachio, Template_relevance Bkms_metabolic, Template_relevance Pistachio_ringbreaker, Template_relevance Reaxys, Template_relevance Reaxys_biocatalysis model, leveraging a vast database of chemical reactions to predict feasible synthetic routes.
One-Step Synthesis Focus: Specifically designed for one-step synthesis, it provides concise and direct routes for your target compounds, streamlining the synthesis process.
Accurate Predictions: Utilizing the extensive PISTACHIO, BKMS_METABOLIC, PISTACHIO_RINGBREAKER, REAXYS, REAXYS_BIOCATALYSIS database, our tool offers high-accuracy predictions, reflecting the latest in chemical research and data.
Strategy Settings
Precursor scoring | Relevance Heuristic |
---|---|
Min. plausibility | 0.01 |
Model | Template_relevance |
Template Set | Pistachio/Bkms_metabolic/Pistachio_ringbreaker/Reaxys/Reaxys_biocatalysis |
Top-N result to add to graph | 6 |
Feasible Synthetic Routes
Disclaimer and Information on In-Vitro Research Products
Please be aware that all articles and product information presented on BenchChem are intended solely for informational purposes. The products available for purchase on BenchChem are specifically designed for in-vitro studies, which are conducted outside of living organisms. In-vitro studies, derived from the Latin term "in glass," involve experiments performed in controlled laboratory settings using cells or tissues. It is important to note that these products are not categorized as medicines or drugs, and they have not received approval from the FDA for the prevention, treatment, or cure of any medical condition, ailment, or disease. We must emphasize that any form of bodily introduction of these products into humans or animals is strictly prohibited by law. It is essential to adhere to these guidelines to ensure compliance with legal and ethical standards in research and experimentation.