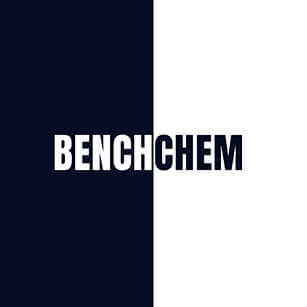
(FERROCENYLMETHYL)TRIMETHYLAMMONIUM BROMIDE
- Click on QUICK INQUIRY to receive a quote from our team of experts.
- With the quality product at a COMPETITIVE price, you can focus more on your research.
Overview
Description
(FERROCENYLMETHYL)TRIMETHYLAMMONIUM BROMIDE is an organic compound containing ammonium and bromide ions. It is commonly used as a phase transfer catalyst in organic synthesis, particularly in the production of pharmaceuticals and agrochemicals . The compound has a strong base that facilitates the transfer of reactants between immiscible solvents, making chemical reactions more efficient .
Mechanism of Action
Target of Action
This compound is a biochemical reagent and can be used as a biological material or organic compound for life science related research .
Mode of Action
It’s known that the compound undergoes a first-order paraelectric–ferroelectric phase transition at the curie temperature (tc) around 286 k, accompanied by significant changes in the structural, thermal, and electrical properties .
Pharmacokinetics
Its solubility in methanol is reported to be very faint , which could potentially impact its bioavailability.
Result of Action
The compound’s significant changes in structural, thermal, and electrical properties at the Curie temperature suggest it may have interesting effects at the molecular level .
Action Environment
Given its phase transition at a specific temperature , it’s reasonable to assume that temperature could be a significant environmental factor.
Biochemical Analysis
Biochemical Properties
(FERROCENYLMETHYL)TRIMETHYLAMMONIUM BROMIDE plays a significant role in biochemical reactions due to its ability to interact with a variety of enzymes, proteins, and other biomolecules. This compound is known to act as an inhibitor in certain biochemical assays, affecting the activity of specific enzymes . The nature of these interactions often involves binding to the active sites of enzymes, thereby altering their activity and influencing the overall biochemical pathways .
Cellular Effects
The effects of this compound on various types of cells and cellular processes are profound. This compound has been shown to influence cell function by affecting cell signaling pathways, gene expression, and cellular metabolism . For instance, it can modulate the activity of key signaling molecules, leading to changes in cellular responses and metabolic activities . Additionally, this compound can impact gene expression by interacting with transcription factors and other regulatory proteins .
Molecular Mechanism
At the molecular level, this compound exerts its effects through various mechanisms. One of the primary mechanisms involves binding interactions with biomolecules, such as enzymes and proteins . This binding can lead to enzyme inhibition or activation, depending on the specific context of the interaction . Furthermore, this compound can induce changes in gene expression by interacting with DNA or RNA molecules, thereby influencing the transcriptional and translational processes .
Temporal Effects in Laboratory Settings
The effects of this compound can change over time in laboratory settings. This compound is known for its stability under specific storage conditions, but it can degrade over time if not stored properly . Long-term studies have shown that this compound can have lasting effects on cellular function, including sustained changes in enzyme activity and gene expression . These temporal effects are crucial for understanding the long-term implications of using this compound in biochemical research .
Dosage Effects in Animal Models
In animal models, the effects of this compound vary with different dosages. At lower doses, this compound can modulate enzyme activity and cellular responses without causing significant toxicity . At higher doses, this compound can exhibit toxic effects, including cellular damage and adverse physiological responses . Understanding the dosage effects is essential for determining the safe and effective use of this compound in experimental settings .
Metabolic Pathways
This compound is involved in various metabolic pathways, interacting with enzymes and cofactors that regulate metabolic flux and metabolite levels . This compound can influence the activity of key metabolic enzymes, thereby altering the flow of metabolites through different pathways . These interactions are critical for understanding the metabolic impact of this compound in biological systems .
Transport and Distribution
Within cells and tissues, this compound is transported and distributed through specific transporters and binding proteins . These interactions facilitate the localization and accumulation of the compound in particular cellular compartments . The transport and distribution of this compound are essential for its biological activity and function .
Subcellular Localization
The subcellular localization of this compound is influenced by targeting signals and post-translational modifications that direct it to specific compartments or organelles . This localization is crucial for the compound’s activity and function, as it determines the specific sites of interaction with biomolecules . Understanding the subcellular localization of this compound is vital for elucidating its role in cellular processes .
Preparation Methods
Synthetic Routes and Reaction Conditions
(FERROCENYLMETHYL)TRIMETHYLAMMONIUM BROMIDE can be synthesized through the reaction of ferrocene with trimethylamine and methyl bromide. The reaction typically involves the following steps:
Ferrocene: reacts with in the presence of a base to form .
Ferrocenylmethyl bromide: then reacts with trimethylamine to yield this compound.
Industrial Production Methods
Industrial production methods for this compound are similar to laboratory synthesis but are scaled up to accommodate larger quantities. The reaction conditions are optimized to ensure high yield and purity of the final product .
Chemical Reactions Analysis
Types of Reactions
(FERROCENYLMETHYL)TRIMETHYLAMMONIUM BROMIDE undergoes various types of chemical reactions, including:
Oxidation: The ferrocene moiety can be oxidized to form ferrocenium ions.
Reduction: The compound can be reduced back to ferrocene from ferrocenium ions.
Substitution: The bromide ion can be substituted with other nucleophiles.
Common Reagents and Conditions
Oxidation: Common oxidizing agents include ferric chloride (FeCl3) and ceric ammonium nitrate (CAN).
Reduction: Reducing agents such as sodium borohydride (NaBH4) are used.
Substitution: Nucleophiles like hydroxide ions (OH-) or cyanide ions (CN-) can be used for substitution reactions.
Major Products
Oxidation: Ferrocenium salts.
Reduction: Ferrocene.
Substitution: Various substituted ferrocenylmethyl derivatives.
Scientific Research Applications
(FERROCENYLMETHYL)TRIMETHYLAMMONIUM BROMIDE has a wide range of applications in scientific research:
Chemistry: Used as a phase transfer catalyst to facilitate reactions between immiscible solvents.
Biology: Employed in biochemical assays and as a reagent in life science research.
Medicine: Utilized in the synthesis of pharmaceutical compounds.
Industry: Applied in the production of agrochemicals and other industrial chemicals.
Comparison with Similar Compounds
Similar Compounds
- (FERROCENYLMETHYL)TRIMETHYLAMMONIUM CHLORIDE
- (FERROCENYLMETHYL)TRIMETHYLAMMONIUM IODIDE
- (FERROCENYLMETHYL)TRIMETHYLAMMONIUM FLUORIDE
Uniqueness
(FERROCENYLMETHYL)TRIMETHYLAMMONIUM BROMIDE is unique due to its strong base properties and its ability to act as an efficient phase transfer catalyst. Compared to its chloride, iodide, and fluoride counterparts, the bromide ion provides optimal balance between reactivity and stability, making it a preferred choice in many synthetic applications .
Properties
CAS No. |
106157-30-6 |
---|---|
Molecular Formula |
C14H20BrFeN |
Molecular Weight |
338.06 g/mol |
IUPAC Name |
cyclopenta-1,3-diene;cyclopenta-1,3-dien-1-ylmethyl(trimethyl)azanium;iron(2+);bromide |
InChI |
InChI=1S/C9H15N.C5H5.BrH.Fe/c1-10(2,3)8-9-6-4-5-7-9;1-2-4-5-3-1;;/h4-7H,8H2,1-3H3;1-5H;1H;/q;-1;;+2/p-1 |
InChI Key |
QNUPENXMUJPLDQ-UHFFFAOYSA-M |
SMILES |
C[N+](C)(C)C[C-]1C=CC=C1.[CH-]1C=CC=C1.[Fe+2].[Br-] |
Canonical SMILES |
C[N+](C)(C)CC1=CC=C[CH-]1.[CH-]1C=CC=C1.[Fe+2].[Br-] |
Origin of Product |
United States |
Q1: How does FcTAB help in understanding the behavior of fluorocarbon surfactants?
A1: FcTAB acts as an electrochemical probe in studying fluorocarbon surfactant solutions []. Due to its positive charge, FcTAB interacts with negatively charged micelles formed by these surfactants. By measuring the diffusion coefficient of FcTAB using cyclic voltammetry, researchers can infer information about the micelle size and shape. For example, a decrease in FcTAB diffusion coefficient indicates the formation of larger aggregates [].
Q2: Can you elaborate on the use of FcTAB in studying ion pair extraction kinetics?
A2: FcTAB, paired with sodium hexafluorophosphate (NaPF6), forms an extractable ion pair that partitions between water and an oil phase (tributyl phosphate) [, ]. By injecting a microdroplet of the oil phase into a solution containing FcTAB and monitoring its electrochemical behavior, researchers can investigate the kinetics of this extraction process. The initial extraction rate, determined electrochemically, provides insights into the rate-limiting step of ion transfer across the oil/water interface [].
Q3: Beyond fluorocarbon surfactants, are there other applications of FcTAB in material science?
A3: Yes, FcTAB has been explored in the development of glucose sensors []. It can be co-immobilized with glucose oxidase within a polypyrrole/poly(styrenesulfonate) film electropolymerized on an electrode surface. The FcTAB acts as a mediator, facilitating electron transfer between the enzyme's active site and the electrode, enabling glucose detection without the need for a soluble mediator [].
Disclaimer and Information on In-Vitro Research Products
Please be aware that all articles and product information presented on BenchChem are intended solely for informational purposes. The products available for purchase on BenchChem are specifically designed for in-vitro studies, which are conducted outside of living organisms. In-vitro studies, derived from the Latin term "in glass," involve experiments performed in controlled laboratory settings using cells or tissues. It is important to note that these products are not categorized as medicines or drugs, and they have not received approval from the FDA for the prevention, treatment, or cure of any medical condition, ailment, or disease. We must emphasize that any form of bodily introduction of these products into humans or animals is strictly prohibited by law. It is essential to adhere to these guidelines to ensure compliance with legal and ethical standards in research and experimentation.