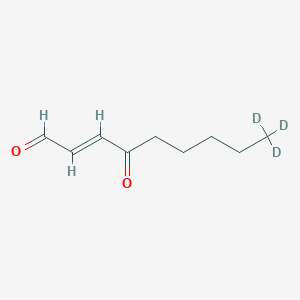
4-oxo-2-Nonenal-d3
Overview
Description
4-Oxo-2-nonenal-d3 (4-ONE-d3) is a deuterated isotopologue of 4-oxo-2-nonenal (4-ONE), a lipid peroxidation product derived from ω-6 polyunsaturated fatty acids such as linoleic acid . The compound features three deuterium atoms at the terminal methyl group, making it chemically identical to 4-ONE except for its isotopic labeling. This deuterium substitution allows 4-ONE-d3 to serve as an internal standard in gas chromatography (GC) or liquid chromatography-mass spectrometry (LC-MS) for precise quantification of endogenous 4-ONE in biological samples .
4-ONE itself is a reactive α,β-unsaturated aldehyde with a ketone group at the C4 position. It modifies proteins (e.g., histidine and lysine residues) and DNA (e.g., 2’-deoxyguanosine), contributing to oxidative stress-related pathologies such as mutagenesis and carcinogenesis .
Preparation Methods
Synthetic Routes and Reaction Conditions: The synthesis of 4-oxo 2-Nonenal-d3 involves the incorporation of deuterium atoms at specific positions in the molecule. This is typically achieved through the hydrogenation of 4-oxo 2-Nonenal using deuterium gas under controlled conditions . The reaction is carried out in the presence of a catalyst, such as palladium on carbon, at elevated temperatures and pressures to ensure complete deuteration.
Industrial Production Methods: Industrial production of 4-oxo 2-Nonenal-d3 follows similar synthetic routes but on a larger scale. The process involves the use of high-purity deuterium gas and advanced catalytic systems to achieve high yields and purity levels. The product is then purified through distillation and chromatography to remove any impurities .
Chemical Reactions Analysis
Types of Reactions: 4-oxo 2-Nonenal-d3 undergoes various chemical reactions, including:
Oxidation: It can be further oxidized to form carboxylic acids.
Reduction: It can be reduced to form alcohols.
Substitution: It can undergo nucleophilic substitution reactions with amines and thiols.
Common Reagents and Conditions:
Oxidation: Common oxidizing agents include potassium permanganate and chromium trioxide.
Reduction: Common reducing agents include sodium borohydride and lithium aluminum hydride.
Substitution: Reactions with amines and thiols typically occur under mild conditions with the use of catalysts such as triethylamine.
Major Products Formed:
Oxidation: Carboxylic acids.
Reduction: Alcohols.
Substitution: Amine and thiol derivatives.
Scientific Research Applications
Biochemical Research
Protein Modification Studies
4-Oxo-2-Nonenal-d3 is primarily used to investigate the modification of proteins through lipid peroxidation. It reacts with amino acids such as histidine and lysine, leading to the formation of adducts that can alter protein function. This property has been exploited in studies examining the effects of oxidative stress on cellular proteins and their roles in various diseases, including neurodegenerative disorders and cardiovascular diseases .
Case Study: HDL Dysfunction
Research indicates that this compound contributes to high-density lipoprotein (HDL) dysfunction, which is linked to atherosclerosis. Elevated levels of 4-oxo-2-nonenal adducts in HDL have been observed in individuals with familial hypercholesterolemia, highlighting its potential role as a biomarker for cardiovascular risk . This finding underscores the importance of studying lipid peroxidation products in understanding metabolic and cardiovascular health.
Toxicology and Pathophysiology
Role in Disease Mechanisms
The compound has been implicated in various pathological conditions due to its ability to induce oxidative stress. For example, studies have shown that exposure to this compound can lead to cellular apoptosis and necrosis, particularly in neuronal cells, which is critical for understanding neurodegenerative diseases like Alzheimer's and Parkinson's .
Case Study: Oocyte Quality
In reproductive biology, research has demonstrated that this compound affects oocyte quality by increasing reactive oxygen species (ROS) levels and causing meiotic abnormalities. This suggests that oxidative stress mediated by lipid peroxidation products can compromise fertility, particularly in aged females .
Pharmaceutical Applications
Potential Therapeutic Targets
Given its role in modifying proteins involved in cell signaling and apoptosis, this compound presents opportunities for therapeutic intervention. Compounds that can mitigate the effects of lipid peroxidation products are being explored as potential treatments for conditions associated with oxidative stress .
Antioxidant Studies
The therapeutic potential of antioxidants to counteract the effects of this compound has been investigated. For instance, co-treatment with antioxidants has shown promise in restoring oocyte quality compromised by oxidative stress . This highlights the potential for developing antioxidant therapies targeting lipid peroxidation pathways.
Analytical Chemistry
Quantification Techniques
The quantification of this compound in biological samples is crucial for understanding its role in health and disease. Advanced analytical techniques such as mass spectrometry have been employed to measure levels of this compound accurately. These methods enable researchers to correlate oxidative stress markers with disease states effectively .
Summary Table of Applications
Mechanism of Action
4-oxo 2-Nonenal-d3 exerts its effects through the modification of proteins and nucleic acids. It reacts with histidine and lysine residues on proteins, leading to protein cross-linking and the formation of advanced glycation end-products . It also modifies 2’-deoxyguanosine in DNA, implicating lipid peroxidation in mutagenesis and carcinogenesis . The compound’s cytotoxicity is attributed to its ability to disrupt cellular membranes and induce oxidative stress .
Comparison with Similar Compounds
4-Oxo-2-Nonenal (4-ONE)
- Structure : C₉H₁₄O₂; α,β-unsaturated aldehyde with a ketone at C3.
- Reactivity: Forms stable 4-ketoamide adducts with lysine residues via intramolecular cyclization, rather than Michael adducts as previously assumed . This reactivity distinguishes it from other lipid peroxidation products like 4-hydroxy-2-nonenal (HNE).
- Biological Role : Induces protein cross-linking, DNA modification, and cytotoxicity. Used as a biomarker for lipid peroxidation .
- Analytical Use : Quantified via LC-MS using 4-ONE-d3 as an internal standard .
4-Hydroxy-2-Nonenal (HNE)
- Structure : C₉H₁₆O₂; α,β-unsaturated aldehyde with a hydroxyl group at C4.
- Reactivity: Forms Michael adducts with cysteine, histidine, and lysine residues.
- Biological Role : Associated with inflammation, apoptosis, and neurodegenerative diseases. Less reactive than 4-ONE but more stable in aqueous environments .
- Isomerism: 4-Oxo-2-nonenol (ONO), the reduced form of 4-ONE, is isomeric with HNE, complicating adduct attribution in biological studies .
(E)-4-Oxohex-2-enal
- Structure : C₆H₈O₂; shorter-chain analog of 4-ONE with similar α,β-unsaturated aldehyde and ketone groups.
- Reactivity : Exhibits analogous electrophilic properties but reduced steric hindrance due to its shorter carbon chain. Forms adducts with glutathione (GSH) and proteins, though less studied than 4-ONE .
- Applications : Used in model studies to explore the reactivity of α,β-unsaturated aldehydes in smaller systems .
4-Oxododecanedioic Acid
- Structure : C₁₂H₂₀O₅; dicarboxylic acid with a ketone group.
- Reactivity: Lacks the α,β-unsaturated aldehyde motif, rendering it non-reactive toward nucleophilic residues like lysine or cysteine. Primarily involved in metabolic pathways rather than oxidative stress .
4-Oxo-2-Nonenol (ONO)
- Structure : C₉H₁₆O₂; reduced alcohol form of 4-ONE.
- Reactivity : Forms GSH adducts similar to HNE but derived from 4-ONE reduction. Challenges the specificity of adduct attribution in studies using HNE .
- Biological Role: Highlights the need for re-evaluation of HNE-specific biomarkers due to isomerism with ONO .
Data Table: Structural and Functional Comparison
Key Research Findings
Adduct Specificity : 4-ONE forms unique 4-ketoamide adducts with lysine residues, unlike HNE, which forms Michael adducts. This distinction is critical for accurate biomarker identification in oxidative stress studies .
Isomerism Challenges: ONO, the reduced form of 4-ONE, is isomeric with HNE, necessitating re-evaluation of adducts previously attributed solely to HNE .
Analytical Utility : 4-ONE-d3’s deuterium labeling ensures minimal interference in mass spectrometry, enabling precise quantification of 4-ONE in complex matrices .
Reactivity Hierarchy : 4-ONE is more electrophilic than HNE due to its ketone group, leading to faster adduct formation and greater protein/DNA damage .
Biological Activity
4-Oxo-2-nonenal-d3 (ONE-d3) is a lipid peroxidation product derived from the oxidative degradation of polyunsaturated fatty acids, particularly linoleic acid. It is a highly reactive electrophile that can form adducts with proteins, DNA, and other biomolecules, leading to various biological effects. This article explores the biological activity of ONE-d3, focusing on its mechanisms of action, toxicity, and implications in disease processes.
Mechanisms of Formation and Reactivity
This compound is generated through the oxidation of n-6 polyunsaturated fatty acids. The oxidative stress environment enhances its formation, leading to increased levels in tissues under pathological conditions. The reactivity of ONE-d3 is primarily attributed to its ability to form covalent bonds with nucleophilic sites on proteins and nucleic acids.
Key Reactions
- Michael Addition : ONE-d3 can undergo Michael addition with thiol and amine groups in proteins, leading to the modification of amino acid residues.
- Schiff Base Formation : It readily forms Schiff bases with lysine residues, which can alter protein function and stability .
Cytotoxicity
Research indicates that ONE-d3 exhibits significant cytotoxic effects, particularly in neuronal cells. In vitro studies have shown that it is 4-5 times more neurotoxic than its analog, 4-hydroxy-2-nonenal (HNE), at low micromolar concentrations. This increased neurotoxicity is attributed to its higher reactivity with cellular proteins .
Table 1: Comparative Neurotoxicity of Aldehydes
Compound | Neurotoxicity (relative to HNE) | Concentration (µM) |
---|---|---|
4-Oxo-2-nonenal | 4-5 times higher | Low micromolar |
4-Hydroxy-2-nonenal | Baseline | Low micromolar |
Protein Modification and Dysfunction
ONE-d3 modifies proteins such as apolipoprotein A-I (apoA-I) in high-density lipoproteins (HDL), leading to HDL dysfunction. Elevated levels of ONE-d3 adducts have been observed in individuals with familial hypercholesterolemia, contributing to atherogenesis by impairing HDL's protective functions against inflammation .
Case Study: HDL Dysfunction
A study found that individuals with familial hypercholesterolemia had significantly higher levels of ONE-d3 adducts in HDL compared to healthy controls (54.6 ± 33.8 pmol/mg vs. 15.3 ± 5.6 pmol/mg) . This suggests that the modification of HDL by ONE-d3 plays a crucial role in cardiovascular diseases.
Obesity and Metabolic Disorders
ONE-d3 has been implicated in obesity-related metabolic disorders. High-fat diets increase the levels of lipid peroxidation products, including ONE-d3, which may contribute to insulin resistance and other metabolic dysfunctions through oxidative stress mechanisms .
Neurodegenerative Diseases
The neurotoxic properties of ONE-d3 position it as a potential contributor to neurodegenerative diseases such as Alzheimer's and Parkinson's disease. Its ability to induce protein aggregation and modify neuronal proteins suggests a role in disease pathogenesis through mechanisms involving oxidative damage and inflammation .
Q & A
Q. How is 4-oxo-2-Nonenal-d3 synthesized and characterized in laboratory settings?
Basic Research Focus
this compound is synthesized via deuterium incorporation at specific positions, typically using deuterated precursors (e.g., deuterium oxide or deuterated aldehydes) in a controlled reaction environment. Key steps include:
- Deuterium labeling : Employing acid- or base-catalyzed exchange reactions to introduce deuterium at the C-2 position .
- Purification : High-performance liquid chromatography (HPLC) or column chromatography to isolate the compound from byproducts.
- Characterization : Nuclear magnetic resonance (NMR) spectroscopy (e.g., H-NMR) and high-resolution mass spectrometry (HRMS) to confirm isotopic purity and structural integrity .
Q. What analytical techniques are most effective for detecting this compound in complex biological matrices?
Basic Research Focus
Liquid chromatography-tandem mass spectrometry (LC-MS/MS) is the gold standard due to its sensitivity and specificity. Methodological considerations include:
- Chromatographic separation : Reverse-phase columns (C18) with gradient elution to resolve this compound from endogenous lipids.
- Mass spectrometry : Multiple reaction monitoring (MRM) targeting deuterium-specific fragmentation patterns to distinguish it from non-deuterated analogs .
- Internal standardization : Co-injection with non-deuterated 4-oxo-2-Nonenal for quantification accuracy.
Q. How can isotopic effects of deuterium in this compound influence experimental outcomes in kinetic studies?
Advanced Research Focus
Deuterium’s higher mass reduces bond vibration frequencies, potentially altering reaction kinetics (kinetic isotope effect, KIE). To mitigate bias:
- Control experiments : Compare reaction rates of deuterated vs. non-deuterated forms under identical conditions.
- Computational modeling : Use density functional theory (DFT) to predict isotopic effects on transition states.
- Statistical validation : Apply ANOVA to assess significance of isotopic deviations in replicate experiments .
Q. What strategies resolve contradictions in data from studies using this compound as a lipid peroxidation biomarker?
Advanced Research Focus
Contradictions may arise from variability in sample preparation, detection limits, or biological context. Solutions include:
- Standardized protocols : Adopt uniform extraction methods (e.g., solid-phase extraction) and calibration curves.
- Meta-analysis : Pool data from independent studies to identify confounding variables (e.g., cell type, oxidative stress inducers) .
- Cross-validation : Correlate this compound levels with complementary biomarkers (e.g., malondialdehyde) to confirm specificity .
Q. How can researchers design experiments to investigate the metabolic fate of this compound in cellular models?
Advanced Research Focus
- Isotopic tracing : Incubate cells with C-labeled glucose to track incorporation into this compound via lipid peroxidation pathways.
- Knockdown/knockout models : Use CRISPR-Cas9 to silence enzymes (e.g., cytochrome P450s) and assess their role in metabolizing the compound.
- Time-course assays : Measure intracellular concentrations at intervals to model metabolic turnover rates .
Q. What role does this compound play in elucidating oxidative stress mechanisms in neurodegenerative disease models?
Advanced Research Focus
- In vivo imaging : Combine deuterated probes with two-photon microscopy to visualize oxidative damage in neuronal tissues.
- Proteomic profiling : Identify adducts formed between this compound and proteins (e.g., via Michael addition) using affinity purification-MS .
- Dose-response studies : Establish thresholds for toxicity by correlating this compound levels with markers of apoptosis (e.g., caspase-3 activation).
Q. How should researchers address ethical and reproducibility challenges when using this compound in interdisciplinary studies?
Advanced Research Focus
- Data transparency : Share raw LC-MS/MS files and synthetic protocols in public repositories (e.g., Zenodo) to enhance reproducibility .
- Ethical review : For studies involving human-derived samples, ensure Institutional Review Board (IRB) approval and adherence to GDPR guidelines for data anonymization .
- Inter-lab collaboration : Conduct round-robin trials to harmonize analytical workflows across institutions .
Q. What computational tools are recommended for modeling the interaction of this compound with cellular targets?
Advanced Research Focus
- Molecular docking : Use AutoDock Vina to predict binding affinities with receptors like the Keap1-Nrf2 complex.
- Molecular dynamics (MD) simulations : Simulate deuterium’s impact on ligand-receptor stability over nanosecond timescales.
- Machine learning : Train models on existing adduct datasets to predict novel interaction sites .
Properties
IUPAC Name |
(E)-9,9,9-trideuterio-4-oxonon-2-enal | |
---|---|---|
Source | PubChem | |
URL | https://pubchem.ncbi.nlm.nih.gov | |
Description | Data deposited in or computed by PubChem | |
InChI |
InChI=1S/C9H14O2/c1-2-3-4-6-9(11)7-5-8-10/h5,7-8H,2-4,6H2,1H3/b7-5+/i1D3 | |
Source | PubChem | |
URL | https://pubchem.ncbi.nlm.nih.gov | |
Description | Data deposited in or computed by PubChem | |
InChI Key |
SEPPVOUBHWNCAW-PKJLGKQPSA-N | |
Source | PubChem | |
URL | https://pubchem.ncbi.nlm.nih.gov | |
Description | Data deposited in or computed by PubChem | |
Canonical SMILES |
CCCCCC(=O)C=CC=O | |
Source | PubChem | |
URL | https://pubchem.ncbi.nlm.nih.gov | |
Description | Data deposited in or computed by PubChem | |
Isomeric SMILES |
[2H]C([2H])([2H])CCCCC(=O)/C=C/C=O | |
Source | PubChem | |
URL | https://pubchem.ncbi.nlm.nih.gov | |
Description | Data deposited in or computed by PubChem | |
Molecular Formula |
C9H14O2 | |
Source | PubChem | |
URL | https://pubchem.ncbi.nlm.nih.gov | |
Description | Data deposited in or computed by PubChem | |
Molecular Weight |
157.22 g/mol | |
Source | PubChem | |
URL | https://pubchem.ncbi.nlm.nih.gov | |
Description | Data deposited in or computed by PubChem | |
Disclaimer and Information on In-Vitro Research Products
Please be aware that all articles and product information presented on BenchChem are intended solely for informational purposes. The products available for purchase on BenchChem are specifically designed for in-vitro studies, which are conducted outside of living organisms. In-vitro studies, derived from the Latin term "in glass," involve experiments performed in controlled laboratory settings using cells or tissues. It is important to note that these products are not categorized as medicines or drugs, and they have not received approval from the FDA for the prevention, treatment, or cure of any medical condition, ailment, or disease. We must emphasize that any form of bodily introduction of these products into humans or animals is strictly prohibited by law. It is essential to adhere to these guidelines to ensure compliance with legal and ethical standards in research and experimentation.