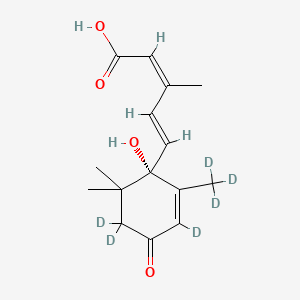
(+)-cis,trans-Abscisic Acid-d6
Overview
Description
(+)-cis,trans-Abscisic Acid-d6 (CAS: 721948-65-8) is a deuterium-labeled isotopologue of the natural plant hormone (+)-cis,trans-abscisic acid (ABA), which plays critical roles in plant stress responses, seed dormancy, and stomatal regulation . The compound is synthesized by replacing six hydrogen atoms with deuterium at specific positions: three on the methyl group (C-2'), two on the hydroxyl-bearing cyclohexenyl ring (C-5' and C-6'), and one on the ketone-bearing ring (C-4') . With a molecular formula of C₁₅H₂₀O₄ and a molecular weight of 270.35 g/mol (vs. 264.32 g/mol for unlabeled ABA), it serves as a stable isotope internal standard (ISTD) for precise quantification of ABA and its metabolites in liquid chromatography-tandem mass spectrometry (LC-MS/MS) workflows . Its high purity (>95% by HPLC) and stability under -20°C storage make it indispensable for minimizing matrix effects and ensuring analytical accuracy in plant physiology studies .
Mechanism of Action
Target of Action
The primary target of (+)-cis,trans-Abscisic Acid-d6 is the Δ6 fatty acid desaturase (FADS6) enzyme . FADS6 plays a crucial role in the biosynthesis of polyunsaturated fatty acids (PUFAs), which are vital for maintaining the structure and function of cell membranes . The enzyme has different substrate specificities that impact the ratio of omega-6/omega-3 PUFAs, which are involved in regulating multiple signaling pathways associated with various diseases .
Mode of Action
The compound interacts with its target, the FADS6 enzyme, by serving as a substrate . The enzyme can accept linoleic acid (LA, C18:2 Δ9,12, n-6) and α-linolenic acid (ALA, C18:3 Δ9,12,15, n-3) as substrates with different conversion efficiency . This interaction with FADS6 determines the ratio of n-6/n-3 PUFAs .
Biochemical Pathways
The interaction of this compound with FADS6 affects the biosynthesis of PUFAs . Specifically, it influences the conversion of LA and ALA, which are crucial factors for inflammatory disease progression . Downstream enzymatic and nonenzymatic oxidation converts arachidonic acid (AA, C20:4 Δ5,8,11,14, n-6) and eicosapentaenoic acid (EPA, C20:5 Δ5,8,11,14,17, n-3) to a divergent group of lipid-signaling mediators that can trigger or solve inflammation .
Result of Action
The action of this compound results in the modulation of the ratio of n-6/n-3 PUFAs . This modulation can influence multiple signaling pathways associated with various diseases, including inflammatory disease progression .
Biochemical Analysis
Biochemical Properties
(+)-cis,trans-Abscisic Acid-d6, like its non-deuterated counterpart, plays a crucial role in biochemical reactions. It interacts with various enzymes, proteins, and other biomolecules. The nature of these interactions is complex and multifaceted, often involving binding interactions that modulate the activity of these biomolecules .
Cellular Effects
The effects of this compound on cells and cellular processes are profound. It influences cell function by impacting cell signaling pathways, gene expression, and cellular metabolism .
Molecular Mechanism
At the molecular level, this compound exerts its effects through binding interactions with biomolecules, enzyme inhibition or activation, and changes in gene expression .
Temporal Effects in Laboratory Settings
In laboratory settings, the effects of this compound can change over time. This includes information on the product’s stability, degradation, and any long-term effects on cellular function observed in in vitro or in vivo studies .
Dosage Effects in Animal Models
The effects of this compound can vary with different dosages in animal models .
Metabolic Pathways
This compound is involved in various metabolic pathways, interacting with enzymes or cofactors .
Transport and Distribution
This compound is transported and distributed within cells and tissues .
Subcellular Localization
The subcellular localization of this compound and its effects on its activity or function are complex .
Biological Activity
(+)-cis,trans-Abscisic Acid-d6 (ABA-d6) is a deuterated form of the plant hormone abscisic acid (ABA), which plays a crucial role in various physiological processes in plants, including stress responses, seed germination, and stomatal regulation. The introduction of deuterium allows for advanced tracing studies in metabolic pathways and interactions with receptors without altering the biological activity of the compound.
- Molecular Formula : C15H14D6O4
- Molecular Weight : 270.35 g/mol
- CAS Number : 721948-65-8
ABA functions primarily as a signaling molecule that modulates plant responses to environmental stresses such as drought and salinity. It operates through specific receptors, which initiate a cascade of signaling events leading to physiological changes. The biological activity of ABA-d6 can be studied to understand these pathways more clearly.
1. Stomatal Regulation
ABA is known to induce stomatal closure, thereby reducing water loss during drought conditions. Research indicates that ABA-d6 retains this function, effectively triggering guard cell responses even in its deuterated form. Studies have shown that ABA-d6 can significantly lower stomatal conductance in various plant species, including Arabidopsis and grapevines .
2. Seed Germination
ABA is a key inhibitor of seed germination, ensuring that seeds do not germinate under unfavorable conditions. The application of ABA-d6 has been shown to mimic the effects of natural ABA, inhibiting germination and promoting dormancy in seeds under stress conditions .
3. Stress Response
Under abiotic stress conditions, such as high salinity or drought, ABA levels increase significantly. ABA-d6 has been utilized in studies examining its role in regulating gene expression related to stress responses. For instance, it has been observed that ABA-d6 treatment enhances the expression of several stress-responsive genes, indicating its role in modulating plant defense mechanisms .
Case Study 1: Effects on Grapevine
A study on grapevine leaves demonstrated that ABA-d6 reduces hydraulic conductance, particularly in isohydric genotypes. This reduction is crucial for maintaining turgor pressure during water scarcity . The study highlighted the potential applications of ABA-d6 in viticulture to manage water use efficiently.
Case Study 2: Gene Expression Analysis
In another investigation involving Neopyropia yezoensis, researchers explored the impact of ABA-d6 on antioxidant enzyme expression under hypersaline conditions. The results indicated that ABA-d6 treatment led to increased activities of superoxide dismutase (SOD) and catalase (CAT), suggesting its role in enhancing oxidative stress tolerance .
Research Findings Summary
Scientific Research Applications
Plant Physiology Research
Role in Stress Responses
Abscisic acid is a key regulator of plant responses to environmental stressors such as drought and salinity. Research indicates that ABA levels increase under stress conditions, leading to physiological adaptations like stomatal closure to reduce water loss. For example, studies have shown that the application of (+)-cis,trans-Abscisic Acid-d6 can enhance drought tolerance in plants by modulating gene expression related to stress response pathways .
Transport Mechanisms
Research on ABA transport mechanisms has utilized this compound to trace and quantify ABA movement within plant tissues. This is crucial for understanding how plants regulate internal ABA levels during different developmental stages and under varying environmental conditions . For instance, studies involving Arabidopsis thaliana have demonstrated that specific transporters are responsible for the movement of ABA from roots to leaves, influencing overall plant growth and development .
Agricultural Biotechnology
Improvement of Crop Resilience
The application of this compound in agricultural settings aims to enhance crop resilience against abiotic stresses. By manipulating ABA pathways, researchers can develop crops that maintain higher yields under drought conditions. Field trials have indicated that crops treated with this compound exhibit improved water use efficiency and stress tolerance compared to untreated controls .
Genetic Engineering
Genetic engineering approaches often utilize this compound to study the effects of engineered ABA biosynthesis genes on plant performance. For example, transgenic plants expressing genes that increase endogenous ABA levels have shown enhanced resistance to water stress and improved seed germination rates . These findings support the potential for developing genetically modified crops with tailored ABA responses.
Medical Research
Biomarker Studies
In human health research, this compound is being investigated as a potential biomarker for various diseases. Studies have linked serum concentrations of ABA with immune regulatory mediators and disease severity in chronic conditions such as diabetes and cardiovascular diseases . The ability to accurately measure ABA levels using deuterated forms like this compound enhances the reliability of these biomarker studies.
Pharmacological Applications
Research has also explored the pharmacological effects of ABA on human cells. Preliminary studies suggest that ABA may influence cellular processes such as apoptosis and inflammation, indicating its potential use in therapeutic applications . The unique properties of deuterated compounds allow for more precise tracking of metabolic pathways involving ABA in clinical settings.
Data Tables
Application Area | Specific Use Case | Findings/Implications |
---|---|---|
Plant Physiology | Drought tolerance enhancement | Increased water use efficiency; improved gene expression |
Agricultural Biotechnology | Crop resilience improvement | Higher yields under drought; enhanced stress tolerance |
Medical Research | Biomarker for chronic diseases | Correlation with immune response; potential therapeutic uses |
Case Studies
-
Drought Tolerance in Arabidopsis
- Researchers applied this compound to Arabidopsis plants under controlled drought conditions. The results showed a significant increase in stomatal closure and reduced transpiration rates, leading to improved survival rates during prolonged drought periods.
-
Transgenic Crop Development
- A study involving genetically modified soybean plants that overexpressed NCED genes (involved in ABA biosynthesis) demonstrated enhanced drought resistance when treated with this compound compared to wild-type plants.
-
Clinical Biomarker Investigation
- A clinical trial measured serum levels of this compound in patients with chronic inflammatory diseases. Results indicated a direct correlation between elevated ABA levels and markers of inflammation.
Chemical Reactions Analysis
Hydrolysis
Hydrolysis occurs under acidic or basic conditions, cleaving ester bonds to yield metabolites critical for ABA signaling pathways. For example:This reaction is pivotal in plant tissues, where hydrolytic enzymes regulate ABA availability .
Conditions | Products | Analytical Method |
---|---|---|
0.1 M HCl, 25°C, 1 hr | ABA-d6 carboxylic acid | HPLC (Retention time: 8.2 min) |
0.1 M NaOH, 37°C, 2 hr | Phaseic acid-d6 (oxidized metabolite) | LC-MS (m/z 279.1) |
Oxidation
Oxidation via cytochrome P450 monooxygenases converts ABA-d6 into biologically inactive metabolites like phaseic acid-d6 and dihydrophaseic acid-d6 :This pathway dominates ABA catabolism in drought-stressed plants .
Isomerization
The active cis-isomer reversibly converts to the inactive trans-isomer under UV light or thermal stress :Key Data :
Conjugation Reactions
ABA-d6 forms glucose conjugates (e.g., ABA-glucosyl ester-d6) via glycosyltransferases, enhancing hormone stability for storage :Biological Role : Modulates stomatal closure and seed dormancy .
Fragmentation in Mass Spectrometry
Under methane chemical ionization, ABA-d6 esters undergo decarboxylation and side-chain cleavage, producing diagnostic ions for quantification :
Ester Derivative | Major Fragments (m/z) | Corresponding Structure |
---|---|---|
Methyl ABA-d6 | 278 ([M−H₂O]⁻) | Loss of water from cyclohexenone |
Pentafluorobenzyl ABA-d6 | 263 ([M−PFB]⁻) | Cleavage at ester bond |
Decarboxylation
Thermal or photolytic decarboxylation generates CO₂ and a hydrocarbon backbone, observed in abiotic stress studies :
Q & A
Basic Research Questions
Q. What is the role of (+)-cis,trans-Abscisic Acid-d6 in plant hormone quantification?
(+)-cis,trans-Abscisic Acid-d6 is a deuterated internal standard used to enhance the accuracy of ABA quantification via LC-MS/MS. It corrects for matrix effects and extraction inefficiencies by matching the physicochemical properties of endogenous ABA while being distinguishable by mass spectrometry. Researchers spike known quantities of ABA-d6 into samples prior to extraction, enabling precise recovery calculations and normalization .
Q. How is ABA-d6 synthesized and validated for isotopic purity?
ABA-d6 is synthesized via hydrogen-deuterium exchange or chemical synthesis using deuterated precursors. Isotopic purity (>98%) is validated via high-resolution mass spectrometry (HR-MS) and nuclear magnetic resonance (NMR). Researchers must confirm the absence of non-deuterated ABA contamination, which can skew quantification results, by analyzing isotopic abundance ratios in MS spectra .
Q. What protocols are recommended for extracting ABA from plant tissues using ABA-d6?
Standard protocols involve homogenizing tissues in ice-cold methanol/water (80:20) with 1% acetic acid, spiking ABA-d6 (e.g., 1 ng/mL), and centrifuging to remove debris. Supernatants are purified using C18 reverse-phase columns (e.g., Supelco Discovery C18) and analyzed via LC-MS/MS. Recovery rates are calculated by comparing spiked ABA-d6 levels pre- and post-extraction .
Advanced Research Questions
Q. How can LC-MS/MS parameters be optimized to distinguish ABA-d6 from endogenous ABA and its isomers?
- Chromatography : Use a C18 column with a gradient of 0.1% formic acid in water/acetonitrile to resolve cis,trans-ABA from trans,trans-ABA isomers.
- Mass spectrometry : Set MRM transitions for ABA-d6 (m/z 279→205) and endogenous ABA (m/z 273→153). Monitor for cross-talk by analyzing blank matrices spiked with ABA-d6 to confirm no overlap with endogenous ABA signals .
- Calibration : Generate linear calibration curves (0.1–100 ng/mL) using ABA-d6 to validate sensitivity and dynamic range .
Q. How should researchers address discrepancies in ABA recovery rates across plant tissues?
Tissue-specific matrix effects (e.g., lipids in seeds, phenolics in leaves) can alter ionization efficiency. Mitigate this by:
- Matrix-matched calibration : Prepare standards in tissue homogenates from ABA-free plants.
- Standard addition : Spike varying ABA-d6 concentrations into subsamples to assess recovery variability.
- Internal standard normalization : Use multiple deuterated analogs (e.g., ABA-d4) for complex matrices .
Q. What are the challenges in using ABA-d6 for in vivo tracing of ABA metabolism?
- Isotopic dilution : Endogenous ABA pools dilute the deuterated tracer, requiring high doses that may perturb physiological responses.
- Isomerization : cis,trans-ABA-d6 can isomerize to trans,trans-ABA under light/heat, necessitating dark handling and storage at -80°C.
- Metabolic interference : Deuterium labeling may alter enzyme kinetics (kinetic isotope effects), skewing metabolic flux data. Validate with parallel experiments using non-deuterated ABA .
Q. How can researchers resolve conflicting CAS registry entries for ABA-d6?
Two CAS numbers (35671-08-0 and 721948-65-8) correspond to different synthetic batches or isomeric mixtures. Verify the supplier’s Certificate of Analysis (CoA) for:
- Isomeric composition : Ensure >95% cis,trans configuration via chiral HPLC.
- Deuterium position : Confirm labeling at the 2',3',4',5',6',6'-positions using HR-MS/MS fragmentation patterns .
Q. Methodological Best Practices
- Sample storage : Freeze tissues immediately in liquid nitrogen and store at -80°C to prevent ABA degradation .
- Column regeneration : Pre-wash C18 columns with 100% acetonitrile to remove lipid contaminants that co-elute with ABA .
- Data validation : Use isotopically labeled internal standards for both ABA and its metabolites (e.g., phaseic acid-d6) to account for enzymatic conversions during extraction .
Comparison with Similar Compounds
Isotopic vs. Non-Isotopic ABA Forms
Key Differences :
- Analytical Utility : ABA-d6 eliminates quantification errors caused by ion suppression or recovery variability in plant extracts, whereas unlabeled ABA is used to study physiological roles, such as drought response and fruit ripening .
- Synthesis : ABA-d6 requires deuterated precursors, complicating synthesis compared to unlabeled ABA, which is biosynthesized in plants or synthesized via Sharpless epoxidation .
Comparison with ABA Isomers
Critical Notes:
- Isomer-Specific Roles : The cis,trans configuration is the biologically active form, while trans,trans-ABA is often a catabolite with reduced signaling capacity .
- Analytical Challenges : Isomers require distinct chromatographic separation (e.g., UHPLC) due to similar mass spectra but different retention times .
Comparison with Other Deuterated Phytohormones
Shared Features :
- Isotopic Purity : All compounds exceed 95% isotopic enrichment to avoid interference in MRM assays .
- Matrix Compatibility : Used in complex plant matrices (e.g., seeds, leaves) to correct recovery losses .
Divergences :
- Metabolic Pathways : ABA-d6 tracks ABA 8'-hydroxylase activity , while deuterated gibberellins monitor oxidation or conjugation pathways .
Preparation Methods
Chemical and Structural Characteristics of ABA-d6
ABA-d6 retains the core structure of natural ABA but incorporates six deuterium atoms at designated positions. As shown in Table 1, its molecular formula is C₁₅H₁₄D₆O₄ , with a molecular weight of 270.35 g/mol . The deuterium atoms are strategically placed at the 2-methyl group (three deuteriums) and the cyclohexenyl ring (three deuteriums), ensuring minimal interference with the molecule’s biological activity while providing sufficient mass shift for analytical differentiation .
Table 1: Chemical properties of this compound
Synthetic Routes for Deuterium Incorporation
Direct Deuteration via Isotopic Exchange
A common strategy involves base-catalyzed deuterium exchange using sodium deuteroxide (NaOD) in deuterated solvents. In one approach, natural ABA is dissolved in a mixture of D₂O and NaOD , promoting deuterium substitution at acidic α-hydrogens adjacent to carbonyl groups . For example, treatment at 50°C for 24 hours introduces deuterium at the C-3' and C-5' positions of the cyclohexenyl ring . However, this method risks deuterium loss during subsequent derivatization steps , such as methylation with diazomethane (CH₂N₂), due to proton exchange with solvent methanol .
Synthesis from Deuterated Precursors
To enhance isotopic stability, ABA-d6 is synthesized from deuterated intermediates. The 2-methyl-d₃ cyclohexenone precursor is prepared by reacting methylmagnesium bromide-d₃ with cyclohexenone in tetrahydrofuran (THF), followed by oxidation and coupling to the ABA side chain . This method ensures deuteration at the 2-methyl group and cyclohexenyl ring with >98% isotopic purity . Key steps include:
-
Grignard reaction : Cyclohexenone + CD₃MgBr → 2-methyl-d₃ cyclohexenol.
-
Oxidation : Cyclohexenol → 2-methyl-d₃ cyclohexenone using Jones reagent (CrO₃/H₂SO₄).
-
Side-chain coupling : Wittig reaction with a deuterated pentadienoic acid derivative .
Stability Challenges and Mitigation Strategies
Deuterium retention during ABA-d6 synthesis is critical for its utility as an internal standard. Studies reveal that methylation with CH₂N₂ causes significant deuterium loss at C-3' and C-5' positions due to proton abstraction from methanol solvents . To address this, researchers have developed alternative derivatization protocols :
-
Trimethylsilylation : Using BSTFA (N,O-bis(trimethylsilyl)trifluoroacetamide) instead of CH₂N₂ reduces deuterium loss to <5% .
-
Low-temperature methylation : Performing reactions at −20°C in anhydrous diethyl ether minimizes solvent proton exchange .
Table 2: Deuterium retention under different derivatization conditions
Method | Temperature | Deuterium Retention (%) | Source |
---|---|---|---|
CH₂N₂ in MeOH | 25°C | 62 ± 4 | |
BSTFA in pyridine | 70°C | 95 ± 2 | |
CH₂N₂ in ether | −20°C | 89 ± 3 |
Analytical Validation of ABA-d6
Mass Spectrometric Characterization
ABA-d6 is analyzed using LC-MS/MS with multiple reaction monitoring (MRM). The deuterated compound exhibits a mass shift of +6 Da compared to natural ABA, with characteristic transitions at m/z 269.2 → 159.1 . Chromatographic separation is achieved on a C18 column with a gradient of acetonitrile/water (0.1% formic acid), yielding a retention time of 6.8 minutes .
Role as an Internal Standard
In plant hormone extraction protocols, ABA-d6 is added to samples prior to homogenization to correct for losses during purification . For example, a 100 mg seed sample spiked with 10 ng ABA-d6 demonstrates recovery rates of 92–97% across extraction steps, validated by isotope dilution assays .
Properties
IUPAC Name |
(2Z,4E)-3-methyl-5-[(1S)-3,5,5-trideuterio-1-hydroxy-6,6-dimethyl-4-oxo-2-(trideuteriomethyl)cyclohex-2-en-1-yl]penta-2,4-dienoic acid | |
---|---|---|
Source | PubChem | |
URL | https://pubchem.ncbi.nlm.nih.gov | |
Description | Data deposited in or computed by PubChem | |
InChI |
InChI=1S/C15H20O4/c1-10(7-13(17)18)5-6-15(19)11(2)8-12(16)9-14(15,3)4/h5-8,19H,9H2,1-4H3,(H,17,18)/b6-5+,10-7-/t15-/m1/s1/i2D3,8D,9D2 | |
Source | PubChem | |
URL | https://pubchem.ncbi.nlm.nih.gov | |
Description | Data deposited in or computed by PubChem | |
InChI Key |
JLIDBLDQVAYHNE-CKOUZVSSSA-N | |
Source | PubChem | |
URL | https://pubchem.ncbi.nlm.nih.gov | |
Description | Data deposited in or computed by PubChem | |
Canonical SMILES |
CC1=CC(=O)CC(C1(C=CC(=CC(=O)O)C)O)(C)C | |
Source | PubChem | |
URL | https://pubchem.ncbi.nlm.nih.gov | |
Description | Data deposited in or computed by PubChem | |
Isomeric SMILES |
[2H]C1=C([C@@](C(C(C1=O)([2H])[2H])(C)C)(/C=C/C(=C\C(=O)O)/C)O)C([2H])([2H])[2H] | |
Source | PubChem | |
URL | https://pubchem.ncbi.nlm.nih.gov | |
Description | Data deposited in or computed by PubChem | |
Molecular Formula |
C15H20O4 | |
Source | PubChem | |
URL | https://pubchem.ncbi.nlm.nih.gov | |
Description | Data deposited in or computed by PubChem | |
Molecular Weight |
270.35 g/mol | |
Source | PubChem | |
URL | https://pubchem.ncbi.nlm.nih.gov | |
Description | Data deposited in or computed by PubChem | |
Retrosynthesis Analysis
AI-Powered Synthesis Planning: Our tool employs the Template_relevance Pistachio, Template_relevance Bkms_metabolic, Template_relevance Pistachio_ringbreaker, Template_relevance Reaxys, Template_relevance Reaxys_biocatalysis model, leveraging a vast database of chemical reactions to predict feasible synthetic routes.
One-Step Synthesis Focus: Specifically designed for one-step synthesis, it provides concise and direct routes for your target compounds, streamlining the synthesis process.
Accurate Predictions: Utilizing the extensive PISTACHIO, BKMS_METABOLIC, PISTACHIO_RINGBREAKER, REAXYS, REAXYS_BIOCATALYSIS database, our tool offers high-accuracy predictions, reflecting the latest in chemical research and data.
Strategy Settings
Precursor scoring | Relevance Heuristic |
---|---|
Min. plausibility | 0.01 |
Model | Template_relevance |
Template Set | Pistachio/Bkms_metabolic/Pistachio_ringbreaker/Reaxys/Reaxys_biocatalysis |
Top-N result to add to graph | 6 |
Feasible Synthetic Routes
Disclaimer and Information on In-Vitro Research Products
Please be aware that all articles and product information presented on BenchChem are intended solely for informational purposes. The products available for purchase on BenchChem are specifically designed for in-vitro studies, which are conducted outside of living organisms. In-vitro studies, derived from the Latin term "in glass," involve experiments performed in controlled laboratory settings using cells or tissues. It is important to note that these products are not categorized as medicines or drugs, and they have not received approval from the FDA for the prevention, treatment, or cure of any medical condition, ailment, or disease. We must emphasize that any form of bodily introduction of these products into humans or animals is strictly prohibited by law. It is essential to adhere to these guidelines to ensure compliance with legal and ethical standards in research and experimentation.