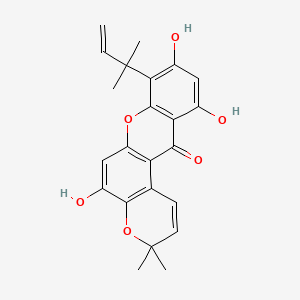
Cudraxanthone B
Overview
Description
Cudraxanthone B (CXB) is a prenylated xanthone compound isolated from the root bark of Cudrania tricuspidata, a plant traditionally used in East Asian medicine for treating inflammation, liver disorders, and tumors . Its molecular formula is C₂₃H₂₂O₆ (molecular weight: 394.42 g/mol), and it is characterized by a xanthone core substituted with isoprenyl groups that enhance its bioactivity .
Preparation Methods
Synthetic Routes and Reaction Conditions
The synthesis of Cudraxanthone B involves several steps, starting from simple aromatic precursors. The key steps include cyclization reactions to form the xanthone core, followed by functional group modifications to introduce the specific substituents present in this compound. Typical reaction conditions involve the use of strong acids or bases, high temperatures, and specific catalysts to facilitate the cyclization and subsequent reactions .
Industrial Production Methods
Industrial production of this compound is primarily based on extraction from natural sources, particularly the roots of Cudrania tricuspidata. The extraction process involves solvent extraction, followed by purification steps such as chromatography to isolate the pure compound. Advances in biotechnological methods, such as plant cell culture, are also being explored to enhance the yield and sustainability of this compound production .
Chemical Reactions Analysis
Types of Reactions
Cudraxanthone B undergoes various chemical reactions, including:
Oxidation: This reaction can introduce additional oxygen-containing functional groups, altering the compound’s properties.
Reduction: Reduction reactions can remove oxygen atoms or reduce double bonds, modifying the compound’s structure.
Common Reagents and Conditions
Common reagents used in these reactions include oxidizing agents like potassium permanganate, reducing agents such as sodium borohydride, and various catalysts to facilitate substitution reactions. Reaction conditions typically involve controlled temperatures, specific solvents, and precise timing to achieve the desired modifications .
Major Products Formed
The major products formed from these reactions depend on the specific reagents and conditions used. For example, oxidation of this compound can yield hydroxylated derivatives, while reduction can produce deoxygenated forms. Substitution reactions can result in a variety of derivatives with different functional groups, enhancing the compound’s versatility for various applications .
Scientific Research Applications
Chemistry: It serves as a valuable intermediate for the synthesis of other xanthone derivatives, which are of interest for their diverse biological activities.
Mechanism of Action
Cudraxanthone B exerts its effects primarily through the inhibition of platelet aggregation. It achieves this by interfering with calcium mobilization and the activation of glycoprotein IIb/IIIa on the platelet surface. This inhibition prevents the binding of fibrinogen and other adhesive molecules, thereby reducing platelet aggregation and clot formation. The compound also affects various signaling pathways, including the phospholipase Cγ2 pathway, which plays a crucial role in platelet activation .
Comparison with Similar Compounds
Xanthones from Cudrania tricuspidata exhibit diverse biological activities, ranging from antitumor to neuroprotective effects. Below is a detailed comparison of CXB with structurally and functionally related xanthones:
Structural Comparison
Key Structural Notes:
- Prenylation (isoprenyl groups) enhances lipophilicity and membrane permeability, critical for bioactivity .
- Catecholic xanthones (e.g., macluraxanthone B) form quinone methides under oxidative conditions, contributing to their antitumor effects .
Antiplatelet Activity
- No cytotoxicity observed at effective doses .
- Other Xanthones: Limited data on antiplatelet effects. Cudraxanthone D and I primarily focus on antitumor and neuroprotective roles .
Antitumor Activity
CXB vs. Others : While CXB lacks reported cytotoxicity, its structural analogs like cudraxanthone I and macluraxanthone B show potent antitumor activity through oxidative stress modulation .
Neuroprotective and Antioxidant Effects
- Cudraxanthone D : Protects against oxygen-glucose deprivation (OGD)-induced neuronal death (EC₅₀: 2.9–34.9 μM) .
- Cudraxanthone C : Exhibits radical-scavenging activity (IC₅₀ for hydroxyl radicals: ~10 μM) via Fe²⁺ chelation .
- CXB: No direct neuroprotective data, suggesting functional divergence due to substituent variations.
Pharmacokinetic and Analytical Data
Biological Activity
Cudraxanthone B is a prenylated xanthone derivative isolated from the plant Cudrania tricuspidata. This compound has garnered attention for its diverse biological activities, including anti-inflammatory, antioxidant, and cytotoxic effects. This article explores the biological activity of this compound, supported by case studies, research findings, and data tables.
1. Anti-inflammatory Effects
This compound exhibits significant anti-inflammatory properties. A study demonstrated that it effectively reduced the production of pro-inflammatory cytokines such as IL-6 and IL-8 in HaCaT cells stimulated by tumor necrosis factor-alpha (TNF-α) and interferon-gamma (IFN-γ). The half-maximal inhibitory concentration (IC50) values for these effects were notably low, indicating high potency.
Cytokine | IC50 (µM) |
---|---|
IL-6 | 11.96 |
IL-8 | 10.29 |
This suggests that this compound could be a potential therapeutic agent in treating inflammatory conditions.
2. Antioxidant Activity
The antioxidant capacity of this compound has been evaluated through various assays. It was found to scavenge free radicals effectively, thereby reducing oxidative stress in cells. This activity is crucial for protecting cells from damage caused by reactive oxygen species (ROS).
3. Cytotoxicity Against Cancer Cells
This compound has demonstrated cytotoxic effects against various cancer cell lines, including breast cancer (MCF-7) and glioblastoma (U87MG). The IC50 values for these cell lines are shown in the table below:
Cell Line | IC50 (µM) |
---|---|
MCF-7 | 22.49 |
U87MG | 15.30 |
These findings indicate that this compound may induce apoptosis in cancer cells, making it a candidate for further cancer research.
The mechanisms underlying the biological activities of this compound involve several pathways:
- Inhibition of NF-κB Signaling : Studies have shown that this compound inhibits the nuclear translocation of NF-κB, a key regulator of inflammation and cell survival.
- Reduction of iNOS and COX-2 Expression : It suppresses the expression of inducible nitric oxide synthase (iNOS) and cyclooxygenase-2 (COX-2), which are critical for inflammatory responses.
Case Studies
Several case studies have been conducted to evaluate the efficacy of this compound:
- Neuroprotective Effects : In a model of neuroinflammation, this compound reduced markers of inflammation in microglial cells stimulated with lipopolysaccharides (LPS), highlighting its potential in treating neurodegenerative diseases.
- Hepatoprotective Activity : Research indicates that this compound may protect liver cells from damage induced by toxins, suggesting its use in liver-related disorders.
Q & A
Basic Research Questions
Q. What are the primary mechanisms by which CXB inhibits collagen-induced platelet aggregation?
CXB suppresses platelet aggregation through three interconnected pathways: (1) inhibition of intracellular calcium mobilization via phosphorylation of IP3 receptor type I (IP3RI) at Ser1756, reducing Ca²⁺ release from the endoplasmic reticulum ; (2) dose-dependent inhibition of thromboxane A2 (TXA2) production by blocking cPLA2 phosphorylation (Ser505) and p38MAPK activation, critical for arachidonic acid metabolism ; and (3) suppression of αIIb/β3 integrin activation, which reduces fibrinogen binding and fibronectin adhesion—key steps in platelet aggregation . These effects were quantified using flow cytometry (fibrinogen binding assays) and ELISA (TXB2 measurement) .
Q. What experimental methodologies are used to assess CXB's antiplatelet effects?
Standard protocols include:
- Platelet aggregation assays : Washed human platelets (10⁸/mL) are preincubated with CXB (10–40 μM) and stimulated with collagen (2.5 μg/mL) or thrombin (0.05 U/mL). Aggregation is measured via light transmission .
- Calcium mobilization : Fura 2-AM fluorescence detects [Ca²⁺]i levels in collagen-stimulated platelets .
- Western blotting : Phosphorylation states of IP3RI, cPLA2, p38MAPK, and VASP are analyzed to confirm signaling modulation .
- Cyclic nucleotide quantification : cAMP and cGMP levels are measured using enzyme immunoassays (EIAs) to evaluate CXB's impact on platelet inhibitory pathways .
Q. How does CXB modulate cyclic nucleotides (cAMP/cGMP) in platelets?
CXB increases cAMP and cGMP levels by 1.5- to 2-fold in collagen-stimulated platelets, likely through inhibition of phosphodiesterase (PDE) activity. Elevated cyclic nucleotides activate protein kinase A (PKA) and G (PKG), which phosphorylate VASP at Ser157/Ser239, inhibiting αIIb/β3 activation . This mechanism mirrors PDE inhibitors like cilostazol but with distinct upstream signaling effects .
Advanced Research Questions
Q. How can contradictory findings on CXB's calcium signaling effects be resolved across studies?
Discrepancies in calcium inhibition efficacy (e.g., partial vs. complete blockade) may arise from differences in:
- Collagen concentrations : Higher agonist doses (e.g., 5 μg/mL vs. 2.5 μg/mL) may overwhelm CXB's inhibitory capacity .
- Pretreatment duration : Shorter preincubation times (e.g., 1 min vs. 3 min) may limit CXB's interaction with IP3RI .
- Platelet preparation : Washed platelets vs. platelet-rich plasma (PRP) can alter CXB bioavailability due to plasma protein binding . Standardizing agonist concentrations and pretreatment protocols across experimental setups is critical for reproducibility.
Q. What strategies optimize CXB's in vitro experimental design to better predict in vivo efficacy?
Key considerations include:
- Physiological relevance : Testing CXB at its IC50 (27.8 μM for collagen-induced aggregation) alongside subtherapeutic doses .
- Dynamic flow conditions : Incorporating microfluidic chambers to simulate shear stress effects on platelet adhesion .
- Combination therapies : Co-administering CXB with low-dose aspirin or P2Y12 inhibitors to assess synergistic effects .
- Species-specific models : Validating results in murine or primate platelets to address interspecies variability in αIIb/β3 activation .
Q. How does CXB compare to other xanthones (e.g., steppogenin, isoderrone) in antiplatelet potency and mechanism?
CXB demonstrates superior inhibition of αIIb/β3 activation (64–97% suppression at 40 μM) compared to steppogenin (40–60%) and isoderrone (30–50%). This difference is attributed to CXB's dual modulation of Akt dephosphorylation and VASP phosphorylation, which are less pronounced in other xanthones . Dose-response curves and fibrin clot retraction assays (67% inhibition for CXB vs. 16% for Y27632 controls) further highlight its efficacy .
Q. What are the limitations of current CXB research in translational applications?
- Lack of in vivo models : Most studies use human platelets ex vivo; animal thrombosis models (e.g., FeCl3-induced arterial injury) are needed to assess CXB's bioavailability and safety .
- Unclear pharmacokinetics : CXB's stability, half-life, and metabolism in plasma remain uncharacterized .
- Off-target effects : High doses (≥40 μM) may non-specifically inhibit kinases beyond p38MAPK and Akt .
Q. Methodological Guidance
Q. How should researchers validate CXB's specificity in signaling pathway inhibition?
- Pharmacological inhibitors : Use selective antagonists (e.g., SB203580 for p38MAPK) alongside CXB to isolate target effects .
- Knockdown/knockout models : CRISPR-Cas9-edited platelets lacking IP3RI or VASP can confirm pathway dependency .
- Dose-response profiling : Compare CXB's IC50 values across multiple pathways (e.g., 27.8 μM for aggregation vs. 18.4 μM for TXA2 inhibition) to identify primary targets .
Q. What statistical approaches are recommended for analyzing CXB's dose-dependent effects?
- ANOVA with Tukey–Kramer post hoc tests : Suitable for comparing multiple CXB concentrations against controls .
- Non-linear regression : Fit dose-response curves to calculate IC50/EC50 values using software like GraphPad Prism .
- Power analysis : Ensure sample sizes (n ≥ 4) are sufficient to detect ≥20% differences in aggregation rates at α = 0.05 .
Q. Data Reproducibility & Transparency
Q. How can researchers address variability in platelet donor samples?
- Pooled platelets : Combine PRP from ≥5 donors to minimize individual variations in αIIb/β3 expression .
- Donor stratification : Exclude samples from individuals using NSAIDs or anticoagulants within 72 hours .
- Batch normalization : Express results as percentage inhibition relative to donor-matched controls .
Properties
IUPAC Name |
5,9,11-trihydroxy-3,3-dimethyl-8-(2-methylbut-3-en-2-yl)pyrano[3,2-a]xanthen-12-one | |
---|---|---|
Source | PubChem | |
URL | https://pubchem.ncbi.nlm.nih.gov | |
Description | Data deposited in or computed by PubChem | |
InChI |
InChI=1S/C23H22O6/c1-6-22(2,3)18-13(25)9-12(24)17-19(27)16-11-7-8-23(4,5)29-20(11)14(26)10-15(16)28-21(17)18/h6-10,24-26H,1H2,2-5H3 | |
Source | PubChem | |
URL | https://pubchem.ncbi.nlm.nih.gov | |
Description | Data deposited in or computed by PubChem | |
InChI Key |
LGKVKWVNUSOTKD-UHFFFAOYSA-N | |
Source | PubChem | |
URL | https://pubchem.ncbi.nlm.nih.gov | |
Description | Data deposited in or computed by PubChem | |
Canonical SMILES |
CC1(C=CC2=C(O1)C(=CC3=C2C(=O)C4=C(O3)C(=C(C=C4O)O)C(C)(C)C=C)O)C | |
Source | PubChem | |
URL | https://pubchem.ncbi.nlm.nih.gov | |
Description | Data deposited in or computed by PubChem | |
Molecular Formula |
C23H22O6 | |
Source | PubChem | |
URL | https://pubchem.ncbi.nlm.nih.gov | |
Description | Data deposited in or computed by PubChem | |
Molecular Weight |
394.4 g/mol | |
Source | PubChem | |
URL | https://pubchem.ncbi.nlm.nih.gov | |
Description | Data deposited in or computed by PubChem | |
Disclaimer and Information on In-Vitro Research Products
Please be aware that all articles and product information presented on BenchChem are intended solely for informational purposes. The products available for purchase on BenchChem are specifically designed for in-vitro studies, which are conducted outside of living organisms. In-vitro studies, derived from the Latin term "in glass," involve experiments performed in controlled laboratory settings using cells or tissues. It is important to note that these products are not categorized as medicines or drugs, and they have not received approval from the FDA for the prevention, treatment, or cure of any medical condition, ailment, or disease. We must emphasize that any form of bodily introduction of these products into humans or animals is strictly prohibited by law. It is essential to adhere to these guidelines to ensure compliance with legal and ethical standards in research and experimentation.