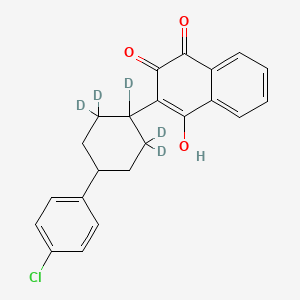
Atovaquone-d5
Overview
Description
Atovaquone-d5 is an organic compound that has been used in a variety of scientific research applications. It is a synthetic derivative of the natural product atovaquone, which is a quinone-methide compound found in the bark of the South American tree, Quassia amara. It has been used in a wide range of studies, ranging from biochemical and physiological research to drug discovery and development.
Scientific Research Applications
Molecular Basis for Atovaquone Binding
Atovaquone inhibits the cytochrome bc1 complex in pathogens like Plasmodium falciparum, Pneumocystis carinii, and Toxoplasma gondii. Research utilizing Atovaquone-d5 could provide detailed insights into its binding mechanism to the bc1 complex. Studies have shown that Atovaquone competitively inhibits the cytochrome bc1 complex, affecting the electron transfer necessary for pathogen respiration. Molecular modeling has elucidated the structural basis for this inhibition, revealing that differences in a single amino acid between species can significantly affect Atovaquone’s efficacy (Kessl et al., 2003).
Atovaquone in Cancer Research
Atovaquone has shown potential in cancer research, particularly in targeting mitochondrial complex III and oxidative phosphorylation (OXPHOS) in cancer stem cells (CSCs). Its repurposing for anti-cancer applications has been explored, with findings indicating that it can inhibit the propagation of CSCs, suggesting a new avenue for therapeutic intervention (Fiorillo et al., 2016).
Understanding Atovaquone Resistance
The emergence of Atovaquone resistance in pathogens is a significant concern. Research into the molecular basis of resistance has highlighted mutations in the cytochrome b gene as a key factor. Modeling resistance in nonpathogenic surrogates like Saccharomyces cerevisiae has provided insights into how these mutations affect Atovaquone binding and pave the way for developing strategies to overcome resistance (Kessl et al., 2007).
Applications in Antiparasitic and Antifungal Research
Atovaquone’s broad-spectrum activity against protozoal infections offers a model for designing new drugs with improved profiles against drug-resistant strains. Studies focusing on the modifications of the hydroxy-naphthoquinone ring of Atovaquone could help in developing compounds less susceptible to resistance, aiding in the treatment of diseases like malaria, toxoplasmosis, and pneumocystis pneumonia (Hughes et al., 2011).
Quality Control in Bioanalytical Assays
The importance of using highly pure internal standards, such as this compound, in liquid chromatography/tandem mass spectrometric (LC/MS/MS) bioanalytical assays has been emphasized. High-purity standards are crucial for accurate quantification and analysis, underscoring the need for rigorous quality control in research involving Atovaquone and its isotopologues (Bergeron et al., 2009).
Mechanism of Action
Target of Action
Atovaquone-d5, a derivative of Atovaquone, primarily targets the cytochrome bc1 complex (Complex III) in the mitochondrial electron transport chain . This complex plays a crucial role in cellular respiration and energy production. This compound also targets other proteins such as PDGFRβ and STAT3 , which are involved in cell proliferation and survival.
Mode of Action
This compound, being a hydroxy-1,4-naphthoquinone analogue of ubiquinone (Co-enzyme Q10), selectively inhibits the mitochondrial electron transport . It interferes with the function of ubiquinone by blocking electron transfer, which disrupts the mitochondrial membrane potential and halts ATP synthesis . This leads to the inhibition of cellular respiration and energy production, causing cell death .
Biochemical Pathways
This compound affects several biochemical pathways. It inhibits the PDGFRβ/NF-κB signaling pathway , leading to the suppression of EMT-related proteins and related inflammatory factors . It also inhibits the HER2/β-Catenin signaling pathway , reducing the expression of downstream molecules such as pGSK-3β, TCF-4, cyclin D1, and c-Myc .
Pharmacokinetics
This compound, like Atovaquone, is highly lipophilic . It exhibits low water solubility, which can lead to variable absorption and bioavailability . Its pharmacokinetics can be improved by formulating it as a prodrug or using other strategies to enhance its solubility . The drug is detected in plasma at concentrations ranging from 250 to 50000 ng/mL .
Result of Action
This compound induces apoptosis and inhibits the growth of cancer cells . It reduces the expression of HER2, β-catenin, and c-Myc, and promotes an increase in apoptosis . It also inhibits oxygen consumption and induces aerobic glycolysis (the Warburg effect), as well as oxidative stress .
Action Environment
The action of this compound can be influenced by environmental factors. For instance, the presence of oxygen can affect the drug’s ability to inhibit oxygen consumption and induce the Warburg effect . Additionally, the drug’s efficacy can be affected by the presence of other drugs or substances in the environment .
Biochemical Analysis
Biochemical Properties
Atovaquone-d5 serves as a versatile reagent in biochemical and physiological studies . Its utility lies in its ability to label proteins and other molecules, allowing for precise tracking and analysis . Acting as a protonophore, this compound holds the unique capability of transporting protons across biological membranes .
Cellular Effects
This compound has been shown to have anticancer effects. It induces apoptosis and inhibits the growth of all the breast cancer cell lines tested, including several patient-derived cells . It also inhibits the expression of E-cadherin protein, and promotes the protein expression of N-cadherin, vimentin, ZEB1, Snail and Slug .
Molecular Mechanism
This compound is thought to act by selectively affecting mitochondrial electron transport and parallel processes such as ATP and pyrimidine biosynthesis in atovaquone-responsive parasites . It also inhibits the expression of NF-κB (p-P65) and related inflammatory factors .
Metabolic Pathways
This compound is thought to affect metabolic pathways by selectively inhibiting mitochondrial electron transport, which in turn affects ATP and pyrimidine biosynthesis .
Transport and Distribution
This compound, being a highly lipophilic drug, is extensively bound to plasma proteins .
Subcellular Localization
This compound is thought to act on the mitochondria due to its ability to inhibit mitochondrial electron transport .
properties
IUPAC Name |
3-[4-(4-chlorophenyl)-1,2,2,6,6-pentadeuteriocyclohexyl]-4-hydroxynaphthalene-1,2-dione | |
---|---|---|
Source | PubChem | |
URL | https://pubchem.ncbi.nlm.nih.gov | |
Description | Data deposited in or computed by PubChem | |
InChI |
InChI=1S/C22H19ClO3/c23-16-11-9-14(10-12-16)13-5-7-15(8-6-13)19-20(24)17-3-1-2-4-18(17)21(25)22(19)26/h1-4,9-13,15,24H,5-8H2/i7D2,8D2,15D | |
Source | PubChem | |
URL | https://pubchem.ncbi.nlm.nih.gov | |
Description | Data deposited in or computed by PubChem | |
InChI Key |
BSJMWHQBCZFXBR-YNUDWXFUSA-N | |
Source | PubChem | |
URL | https://pubchem.ncbi.nlm.nih.gov | |
Description | Data deposited in or computed by PubChem | |
Canonical SMILES |
C1CC(CCC1C2=CC=C(C=C2)Cl)C3=C(C4=CC=CC=C4C(=O)C3=O)O | |
Source | PubChem | |
URL | https://pubchem.ncbi.nlm.nih.gov | |
Description | Data deposited in or computed by PubChem | |
Isomeric SMILES |
[2H]C1(CC(CC(C1([2H])C2=C(C3=CC=CC=C3C(=O)C2=O)O)([2H])[2H])C4=CC=C(C=C4)Cl)[2H] | |
Source | PubChem | |
URL | https://pubchem.ncbi.nlm.nih.gov | |
Description | Data deposited in or computed by PubChem | |
Molecular Formula |
C22H19ClO3 | |
Source | PubChem | |
URL | https://pubchem.ncbi.nlm.nih.gov | |
Description | Data deposited in or computed by PubChem | |
DSSTOX Substance ID |
DTXSID10675642 | |
Record name | 3-[4-(4-Chlorophenyl)(1,2,2,6,6-~2~H_5_)cyclohexyl]-4-hydroxynaphthalene-1,2-dione | |
Source | EPA DSSTox | |
URL | https://comptox.epa.gov/dashboard/DTXSID10675642 | |
Description | DSSTox provides a high quality public chemistry resource for supporting improved predictive toxicology. | |
Molecular Weight |
371.9 g/mol | |
Source | PubChem | |
URL | https://pubchem.ncbi.nlm.nih.gov | |
Description | Data deposited in or computed by PubChem | |
CAS RN |
1217612-80-0 | |
Record name | 3-[4-(4-Chlorophenyl)(1,2,2,6,6-~2~H_5_)cyclohexyl]-4-hydroxynaphthalene-1,2-dione | |
Source | EPA DSSTox | |
URL | https://comptox.epa.gov/dashboard/DTXSID10675642 | |
Description | DSSTox provides a high quality public chemistry resource for supporting improved predictive toxicology. | |
Q & A
Q1: Why is the purity of Atovaquone-d5 critical in bioanalytical methods using LC/MS/MS?
A1: this compound, a deuterated form of Atovaquone, is commonly used as an internal standard (IS) in LC/MS/MS methods for quantifying Atovaquone in biological samples. The research highlights that the presence of impurities, such as this compound to Atovaquone-d8, in the Atovaquone-d4 reference standard can significantly impact method validation []. These impurities may interfere with the accurate quantification of Atovaquone, leading to inaccurate results. Therefore, ensuring high purity of this compound is crucial for reliable and robust bioanalytical method development and validation.
Q2: What steps can be taken to address purity concerns regarding Atovaquone-d4 as an internal standard?
A2: The study emphasizes the importance of scrutinizing the certificate of analysis and even considering recertification of the Atovaquone-d4 standard if the isotopic purity doesn't meet the claimed specifications []. This ensures that the IS used is of acceptable quality and minimizes the risk of impurities interfering with Atovaquone quantification.
Disclaimer and Information on In-Vitro Research Products
Please be aware that all articles and product information presented on BenchChem are intended solely for informational purposes. The products available for purchase on BenchChem are specifically designed for in-vitro studies, which are conducted outside of living organisms. In-vitro studies, derived from the Latin term "in glass," involve experiments performed in controlled laboratory settings using cells or tissues. It is important to note that these products are not categorized as medicines or drugs, and they have not received approval from the FDA for the prevention, treatment, or cure of any medical condition, ailment, or disease. We must emphasize that any form of bodily introduction of these products into humans or animals is strictly prohibited by law. It is essential to adhere to these guidelines to ensure compliance with legal and ethical standards in research and experimentation.