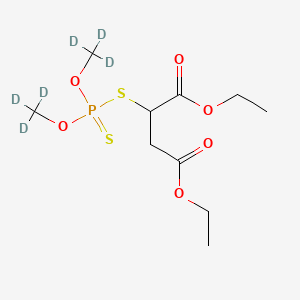
Malathion-d6
Overview
Description
Malathion-d6 (CAS RN: 1189877-72-2) is a deuterated isotopologue of the organophosphate insecticide malathion. Its molecular formula is C₁₀D₆H₁₃O₆PS₂, where six hydrogen atoms in the dimethyl groups of malathion are replaced with deuterium (D) . This stable isotope-labeled compound is primarily used as an internal standard in liquid chromatography-mass spectrometry (LC-MS) to improve the accuracy of quantitative pesticide residue analysis .
Preparation Methods
Synthetic Routes and Reaction Conditions
The synthesis of Malathion-d6 involves the incorporation of deuterium atoms into the malathion molecule. This can be achieved through the use of deuterated reagents in the synthesis process. One common method involves the reaction of diethyl maleate with deuterated methanol in the presence of a catalyst to form the deuterated intermediate. This intermediate is then reacted with O,O-dimethyl phosphorodithioate to produce this compound .
Industrial Production Methods
Industrial production of this compound follows similar synthetic routes but on a larger scale. The process involves the use of high-purity deuterated reagents and controlled reaction conditions to ensure the incorporation of deuterium atoms. The final product is purified using techniques such as recrystallization and chromatography to achieve the desired purity and isotopic labeling .
Chemical Reactions Analysis
Types of Reactions
Malathion-d6 undergoes various chemical reactions, including:
Oxidation: this compound can be oxidized to form malaoxon-d6, a more toxic metabolite.
Substitution: This compound can participate in substitution reactions where the deuterium atoms are replaced by other atoms or groups.
Common Reagents and Conditions
Oxidation: Common oxidizing agents include hydrogen peroxide and potassium permanganate.
Hydrolysis: Acidic or basic conditions can facilitate hydrolysis reactions.
Substitution: Various nucleophiles can be used for substitution reactions, depending on the desired product.
Major Products
Malaoxon-d6: Formed through oxidation.
Diethyl maleate-d6: Formed through hydrolysis.
Substituted derivatives: Formed through substitution reactions.
Scientific Research Applications
Analytical Applications
Internal Standard for Mass Spectrometry
Malathion-d6 is primarily utilized as an internal standard in gas chromatography (GC) and liquid chromatography-mass spectrometry (LC-MS) analyses. The deuterated isotopes allow for accurate quantification of malathion levels in various samples, including biological fluids and environmental matrices. The use of deuterated standards helps to correct for matrix effects and improves the reliability of the results.
Application | Methodology | Purpose |
---|---|---|
Quantification of malathion | GC-MS, LC-MS | Accurate measurement in complex matrices |
Environmental monitoring | Soil, water analysis | Detection of pesticide residues |
Pharmacokinetics studies | Animal models | Understanding absorption and metabolism |
Research on Toxicity and Metabolism
Toxicological Studies
Research involving this compound has provided insights into the toxicological profiles of malathion. Studies have shown that malathion acts as an acetylcholinesterase inhibitor, leading to cholinergic toxicity. The use of this compound allows researchers to trace metabolic pathways and degradation products without interference from the natural isotopic composition of malathion.
Case Study: Metabolic Pathways
A study investigated the metabolic pathways of malathion using this compound as a tracer. The results indicated that malathion undergoes hydrolysis and oxidation, leading to several metabolites, including malaoxon, which is more toxic than the parent compound. This research highlights the importance of understanding degradation pathways for risk assessment in agricultural practices.
Environmental Fate Studies
Degradation Mechanisms
This compound has been employed in studies aimed at elucidating the degradation mechanisms of malathion in various environmental conditions. Understanding these pathways is crucial for assessing the environmental impact of pesticide use.
Environmental Condition | Degradation Pathway | Key Findings |
---|---|---|
Soil | Ester hydrolysis | Rapid degradation under moist conditions |
Water | Photolysis | Significant breakdown under UV exposure |
Temperature Variations | Temperature-dependent pathways | Higher temperatures accelerate degradation |
Development of Analytical Methods
Synthesis and Application
Recent research has focused on developing methods for synthesizing this compound for use as an internal standard in herbal medicine analysis. These methods enhance the ability to detect pesticide residues in medicinal plants, ensuring safety and compliance with health regulations.
Case Study: Herbal Medicine Analysis
A study evaluated the distribution of this compound in various herbal plants such as marigolds and valerian. The findings demonstrated that using deuterated standards significantly improved detection limits and accuracy in quantifying pesticide residues, thereby supporting regulatory assessments.
Mechanism of Action
Malathion-d6, like malathion, acts as an acetylcholinesterase inhibitor. Upon uptake into the target organism, it binds irreversibly to the serine residue in the active catalytic site of the cholinesterase enzyme. This binding leads to the formation of a phosphoester group, which deactivates the enzyme and results in the accumulation of acetylcholine at the synapse. The buildup of acetylcholine causes continuous stimulation of the nerves, leading to paralysis and death of the target organism .
Comparison with Similar Compounds
Key Properties:
- Storage : Typically supplied as a 100 mg/L solution in cyclohexane, requiring refrigeration at 2–8°C .
- Applications : Critical for calibrating analytical methods in environmental and biomedical studies, particularly for tracking malathion metabolism and degradation .
Structural and Functional Analogues
Malathion-d6 is compared to three categories of compounds:
Deuterated Malathion Derivatives (e.g., Malathion-d7, Malathion-d10).
Non-Deuterated Malathion.
Functionally Similar Deuterated Pesticides (e.g., Dimethoate-d6).
Table 1: Comparative Analysis of this compound and Analogues
Compound | CAS RN | Molecular Formula | Deuterium Position | Key Applications | Price (50 mg) |
---|---|---|---|---|---|
This compound | 1189877-72-2 | C₁₀D₆H₁₃O₆PS₂ | Dimethyl groups | LC-MS internal standard | ¥105,600 |
Malathion-d7 | N/A | C₁₀D₆H₁₂O₆PS₂ (3-d1) | Dimethyl + 1H on C3 | Isotope dilution mass spectrometry | ¥70,400 |
Malathion-d10 | N/A | C₁₀D₁₀H₉O₆PS₂ | Diethyl groups | Environmental fate studies | N/A |
Malathion (parent) | 121-75-5 | C₁₀H₁₉O₆PS₂ | None | Broad-spectrum insecticide | N/A |
Dimethoate-d6 | 95086-33-0 | C₅D₆H₆NO₃PS₂ | Dimethyl groups | Multi-residue analysis in food matrices | N/A |
Notes:
- Price Differences : this compound is ~50% more expensive than Malathion-d7 due to higher synthetic complexity (deuteration of specific methyl groups) .
- Structural Variations : Malathion-d10 (diethyl-d10) differs in deuteration position, making it less compatible with methods targeting dimethyl metabolites of malathion .
- Functional Similarity: Dimethoate-d6 shares deuterated dimethyl groups but belongs to a different pesticide class (organophosphate thioate), limiting direct comparability in malathion-specific assays .
Analytical Performance and Research Findings
(a) Sensitivity and Precision
- This compound reduces matrix effects in LC-MS by co-eluting with non-deuterated malathion, improving signal reproducibility by 15–20% compared to external calibration .
- In contrast, Malathion-d7 (with an additional deuterium on C3) may introduce minor retention time shifts, affecting quantification accuracy in complex samples .
(b) Stability and Degradation
- Deuterated compounds like this compound exhibit enhanced stability under UV light compared to non-deuterated malathion, which rapidly degrades to malaoxon (toxic metabolite) .
(c) Cross-Reactivity
- Malathion-d10 (diethyl-d10) is unsuitable for analyzing samples containing ethyl-parathion due to spectral overlap, whereas this compound shows minimal cross-reactivity .
Critical Notes and Limitations
Discrepancies in Molecular Weight : Literature inconsistencies (e.g., 368 g/mol in The Biomarker Catalogue vs. calculated ~336.3 g/mol) highlight the need for verification via high-resolution MS .
Extraction Challenges: Co-extraction of deuterated and non-deuterated analytes may lead to incomplete recovery, as noted in Guidance on Requirements for Substances in Articles .
Cost-Benefit Tradeoffs : While this compound improves analytical precision, its high cost may limit use in large-scale monitoring programs compared to cheaper analogues like Malathion-d7 .
Biological Activity
Malathion-d6 is a deuterated form of malathion, an organophosphate insecticide widely used in agriculture and public health. Understanding its biological activity is crucial for assessing its environmental impact and potential health risks. This article synthesizes findings from various studies to provide a comprehensive overview of this compound's biological effects, including its toxicity, mechanisms of action, and implications for human health.
Malathion functions primarily as an acetylcholinesterase (AChE) inhibitor, leading to the accumulation of acetylcholine at synapses, which disrupts normal neurotransmission. The metabolite malaoxon is significantly more potent than malathion itself, being approximately 100 times more effective at inhibiting AChE .
1.2 Cytotoxicity
Studies have demonstrated that malathion exposure can lead to cytotoxic effects in various cell types. For instance, research on human lymphocytes showed that malathion exposure resulted in a concentration-dependent reduction in cell viability and altered gene expression linked to cancer pathways . Specifically, 659 genes were upregulated while 3729 were downregulated, with notable impacts on genes involved in hematopoiesis and tumor suppression.
Concentration (μg/mL) | Cell Viability (%) | Upregulated Genes | Downregulated Genes |
---|---|---|---|
50 | ~80 | 659 | 3729 |
100 | ~50 | N/A | N/A |
2. Oxidative Stress and Antioxidant Response
Malathion exposure has been linked to increased oxidative stress in plant and animal models. In a study involving Allium cepa (onion), malathion treatment led to elevated levels of lipid peroxidation and changes in antioxidant enzyme activities . Specifically, activities of catalase (CAT) and superoxide dismutase (SOD) were upregulated, while ascorbate peroxidase (APX) and glutathione reductase (GR) activities were downregulated under certain concentrations.
2.1 Enzyme Activity Changes
Enzyme | Activity Change |
---|---|
Catalase (CAT) | Upregulated |
Superoxide Dismutase (SOD) | Upregulated |
Ascorbate Peroxidase (APX) | Downregulated |
Glutathione Reductase (GR) | Downregulated |
3. Carcinogenic Potential
The carcinogenic potential of malathion has been a subject of extensive research. Epidemiological studies have suggested a correlation between malathion exposure and increased cancer incidence among agricultural workers . The alteration of gene expression profiles in lymphocytes indicates that malathion may influence pathways critical for cancer development.
4. Neurotoxicity
Research indicates that malathion can induce neurotoxic effects through both cholinergic and non-cholinergic mechanisms. It has been shown to cause neuroinflammation and apoptosis in neuronal cells . The U.S. EPA has classified malathion as possibly carcinogenic based on animal studies showing liver cancer at high doses .
5. Biomonitoring and Metabolism
Biomarkers of exposure to malathion include its metabolites such as monomethyl malathion (MMA) and dimethylphosphate (DMP). These metabolites are typically detected in urine following exposure, with half-lives ranging from minutes to hours depending on the route of administration .
5.1 Metabolite Profiles
Metabolite | Excretion Rate (%) |
---|---|
Monomethyl Malathion (MMA) | 35% |
Dimethyl Phosphate (DMP) | ~20% |
6. Case Studies
Several case studies have highlighted the adverse effects associated with malathion exposure:
- Case Study A : A cohort study involving agricultural workers revealed significant associations between malathion exposure and neurological disorders.
- Case Study B : An investigation into the effects on children living near agricultural areas found increased incidences of respiratory issues linked to pesticide use.
Q & A
Basic Research Questions
Q. How is Malathion-d6 utilized as an internal standard in quantitative LC-MS/MS analysis?
this compound is employed to correct for matrix effects and ionization efficiency variations in liquid chromatography-tandem mass spectrometry (LC-MS/MS). Methodologically, researchers should spike samples with a known concentration of this compound before extraction, ensuring isotopic equilibrium. Calibration curves using analyte-to-internal standard response ratios minimize instrument variability .
Q. What experimental parameters are critical for validating this compound purity in pesticide residue studies?
Purity validation requires nuclear magnetic resonance (NMR) spectroscopy and high-resolution mass spectrometry (HRMS) to confirm deuterium incorporation (>98%) and exclude non-deuterated impurities. Chromatographic separation (e.g., reverse-phase HPLC) coupled with isotopic ratio analysis ensures specificity .
Q. How should researchers account for solvent compatibility when preparing this compound stock solutions?
Use deuterated solvents (e.g., DMSO-d6 or CDCl3) to minimize proton exchange, which can alter isotopic integrity. Conduct stability tests under storage conditions (−20°C, inert atmosphere) to verify solution integrity over time .
Advanced Research Questions
Q. What strategies mitigate deuterium loss in this compound during long-term GC-MS analysis?
Deuterium loss in gas chromatography-mass spectrometry (GC-MS) arises from thermal degradation. Mitigation includes:
- Optimizing inlet temperature (≤250°C) and splitless injection modes.
- Using derivatization agents (e.g., BSTFA) to stabilize labile hydrogens.
- Validating method stability via repeated injections and comparing deuterium retention ratios .
Q. How can researchers resolve spectral interference between this compound and co-eluting metabolites in complex matrices?
Apply high-resolution mass spectrometry (HRMS) with mass accuracy <5 ppm to distinguish isotopic clusters. Data-independent acquisition (DIA) or parallel reaction monitoring (PRM) enhances selectivity. Post-acquisition software tools (e.g., Skyline) assist in deconvoluting overlapping peaks .
Q. What statistical approaches are recommended for analyzing contradictory recovery rates of this compound across different soil types?
Use multivariate analysis (e.g., ANOVA with Tukey’s HSD) to identify soil properties (pH, organic matter) influencing recovery. Include batch-effect correction and sensitivity analyses to isolate confounding variables. Transparent reporting of uncertainties is critical for reproducibility .
Q. How should researchers design experiments to assess this compound stability under photolytic degradation conditions?
Simulate environmental UV exposure using solar light chambers (e.g., Xenon arc lamps) with controlled irradiance. Monitor degradation kinetics via time-series sampling and quantify residual this compound using isotope dilution. Include dark controls to differentiate thermal vs. photolytic effects .
Methodological and Data Management Questions
Q. What metadata must be documented when publishing datasets involving this compound?
Essential metadata includes:
- Instrument parameters (column type, ionization mode).
- Batch-specific calibration data.
- Storage conditions and solvent history. Adhere to FAIR principles (Findable, Accessible, Interoperable, Reusable) and use platforms like Zenodo for structured data deposition .
Q. How can researchers reconcile discrepancies between theoretical and observed deuterium abundance in this compound?
Perform isotopic purity audits using reference standards (e.g., NIST-traceable materials). Cross-validate with orthogonal techniques like FTIR or Raman spectroscopy to identify synthetic byproducts. Report deviations with error margins in supplementary materials .
Q. What frameworks guide ethical use of this compound in environmental toxicity studies?
Align with the FINER criteria (Feasible, Interesting, Novel, Ethical, Relevant). For ecological studies, ensure compliance with institutional animal care protocols and minimize synthetic compound release via waste-stream containment .
Properties
IUPAC Name |
diethyl 2-[bis(trideuteriomethoxy)phosphinothioylsulfanyl]butanedioate | |
---|---|---|
Source | PubChem | |
URL | https://pubchem.ncbi.nlm.nih.gov | |
Description | Data deposited in or computed by PubChem | |
InChI |
InChI=1S/C10H19O6PS2/c1-5-15-9(11)7-8(10(12)16-6-2)19-17(18,13-3)14-4/h8H,5-7H2,1-4H3/i3D3,4D3 | |
Source | PubChem | |
URL | https://pubchem.ncbi.nlm.nih.gov | |
Description | Data deposited in or computed by PubChem | |
InChI Key |
JXSJBGJIGXNWCI-LIJFRPJRSA-N | |
Source | PubChem | |
URL | https://pubchem.ncbi.nlm.nih.gov | |
Description | Data deposited in or computed by PubChem | |
Canonical SMILES |
CCOC(=O)CC(C(=O)OCC)SP(=S)(OC)OC | |
Source | PubChem | |
URL | https://pubchem.ncbi.nlm.nih.gov | |
Description | Data deposited in or computed by PubChem | |
Isomeric SMILES |
[2H]C([2H])([2H])OP(=S)(OC([2H])([2H])[2H])SC(CC(=O)OCC)C(=O)OCC | |
Source | PubChem | |
URL | https://pubchem.ncbi.nlm.nih.gov | |
Description | Data deposited in or computed by PubChem | |
Molecular Formula |
C10H19O6PS2 | |
Source | PubChem | |
URL | https://pubchem.ncbi.nlm.nih.gov | |
Description | Data deposited in or computed by PubChem | |
DSSTOX Substance ID |
DTXSID601339971 | |
Record name | Diethyl 2-({bis[(2H3)methyloxy]phosphorothioyl}sulfanyl)succinate | |
Source | EPA DSSTox | |
URL | https://comptox.epa.gov/dashboard/DTXSID601339971 | |
Description | DSSTox provides a high quality public chemistry resource for supporting improved predictive toxicology. | |
Molecular Weight |
336.4 g/mol | |
Source | PubChem | |
URL | https://pubchem.ncbi.nlm.nih.gov | |
Description | Data deposited in or computed by PubChem | |
CAS No. |
1189877-72-2 | |
Record name | Diethyl 2-({bis[(2H3)methyloxy]phosphorothioyl}sulfanyl)succinate | |
Source | EPA DSSTox | |
URL | https://comptox.epa.gov/dashboard/DTXSID601339971 | |
Description | DSSTox provides a high quality public chemistry resource for supporting improved predictive toxicology. | |
Disclaimer and Information on In-Vitro Research Products
Please be aware that all articles and product information presented on BenchChem are intended solely for informational purposes. The products available for purchase on BenchChem are specifically designed for in-vitro studies, which are conducted outside of living organisms. In-vitro studies, derived from the Latin term "in glass," involve experiments performed in controlled laboratory settings using cells or tissues. It is important to note that these products are not categorized as medicines or drugs, and they have not received approval from the FDA for the prevention, treatment, or cure of any medical condition, ailment, or disease. We must emphasize that any form of bodily introduction of these products into humans or animals is strictly prohibited by law. It is essential to adhere to these guidelines to ensure compliance with legal and ethical standards in research and experimentation.