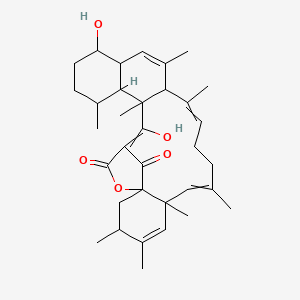
Tetromycin B
Overview
Description
Mechanism of Action
Target of Action
Tetromycin B, like other tetracycline antibiotics, primarily targets the bacterial 30S ribosomal subunit . This subunit is a crucial component of the bacterial protein synthesis machinery. By binding to this target, this compound can effectively inhibit protein synthesis in bacteria, thereby preventing their growth and proliferation .
Mode of Action
This compound exerts its antibacterial effect by binding reversibly to the 30S ribosomal subunit of bacteria . This binding prevents the attachment of aminoacyl tRNA to the mRNA-ribosome complex during translation, thereby disrupting protein synthesis . It also binds to some extent to the bacterial 50S ribosomal subunit and may alter the cytoplasmic membrane, causing intracellular components to leak from bacterial cells .
Biochemical Pathways
The inhibition of protein synthesis by this compound affects multiple biochemical pathways within the bacterial cell. Since proteins serve as enzymes, structural components, and regulators of cellular functions, their synthesis is critical for the survival and growth of bacteria. By disrupting protein synthesis, this compound can interfere with various cellular processes, leading to the death of the bacteria .
Pharmacokinetics
It is known that tetracyclines, in general, are absorbed orally and widely distributed in the body . They are also known to bind to proteins in serum, which can affect their half-life and bioavailability .
Result of Action
The primary result of this compound’s action is the inhibition of bacterial growth. By disrupting protein synthesis, this compound prevents bacteria from carrying out essential cellular functions, leading to their death . This makes this compound effective against a wide range of bacterial infections.
Action Environment
The action of this compound can be influenced by various environmental factors. For instance, the pH of the environment can affect the drug’s solubility and stability, thereby influencing its efficacy . Additionally, the presence of certain ions or compounds can interfere with the drug’s activity. For example, divalent cations such as calcium and magnesium can form chelates with tetracyclines, reducing their antibacterial activity .
Biochemical Analysis
Biochemical Properties
Tetromycin B is a cysteine protease inhibitor, interacting with several enzymes such as rhodesain, falcipain-2, cathepsin L, and cathepsin B . The nature of these interactions is inhibitory, with Ki values in the low micromolar range .
Cellular Effects
This compound has been shown to inhibit the growth of Trypanosoma brucei in vitro . It also exhibits cytotoxicity to HEK293T kidney cells and J774.1 macrophages .
Molecular Mechanism
The mechanism of action of this compound is primarily through its inhibitory effects on cysteine proteases. It binds to these enzymes, preventing them from carrying out their normal function .
Temporal Effects in Laboratory Settings
In laboratory settings, this compound has been shown to have long-lasting effects. For instance, a new this compound derivative, 12-decarboxy-12-methyl tetramycin B, was generated with a titer of 371 ± 26 mg/L through inactivation of a P450 monooxygenase gene tetrG . This derivative exhibited higher antifungal activity against Saccharomyces cerevisiae and Rhodotorula glutinis, but lower hemolytic toxicity to erythrocyte .
Metabolic Pathways
This compound is part of the biosynthetic pathway of polyene antibiotics, which are assembled by multifunctional type I PKSs with acyl-CoA precursors (acetate, propionate, butyrate, etc.) in a sequential set of Claisen-type condensation .
Preparation Methods
Synthetic Routes and Reaction Conditions: The synthesis of Omoconazole involves the reaction of 2,4-dichlorophenylacetonitrile with 4-chlorophenol in the presence of a base to form an intermediate. This intermediate is then reacted with imidazole under specific conditions to yield Omoconazole .
Industrial Production Methods: Industrial production methods for Omoconazole typically involve large-scale synthesis using the same chemical reactions but optimized for higher yields and purity. The process includes purification steps such as recrystallization and chromatography to ensure the final product meets pharmaceutical standards .
Chemical Reactions Analysis
Types of Reactions: Omoconazole undergoes various chemical reactions, including:
Oxidation: Omoconazole can be oxidized under specific conditions to form different metabolites.
Reduction: Reduction reactions can modify the imidazole ring, affecting its antifungal activity.
Common Reagents and Conditions:
Oxidation: Common oxidizing agents include hydrogen peroxide and potassium permanganate.
Reduction: Reducing agents such as lithium aluminum hydride are used.
Substitution: Nucleophilic substitution reactions often involve reagents like sodium hydroxide or potassium carbonate.
Major Products Formed: The major products formed from these reactions include various metabolites and derivatives of Omoconazole, which may have different antifungal properties .
Scientific Research Applications
Omoconazole has several scientific research applications, including:
Chemistry: Used as a model compound to study the synthesis and reactions of azole antifungals.
Biology: Investigated for its effects on fungal cell membranes and its potential to inhibit fungal growth.
Medicine: Used in clinical studies to evaluate its efficacy and safety in treating fungal infections.
Industry: Employed in the development of new antifungal formulations and treatments
Comparison with Similar Compounds
Miconazole: Another azole antifungal with a similar mechanism of action but different chemical structure.
Clotrimazole: Used to treat similar fungal infections but has different pharmacokinetic properties.
Ketoconazole: Known for its broad-spectrum antifungal activity but has more significant side effects compared to Omoconazole
Uniqueness: Omoconazole is unique due to its specific chemical structure, which provides a balance between efficacy and safety. Its ability to inhibit lanosterol 14α-demethylase effectively makes it a valuable antifungal agent with fewer side effects compared to some other azole antifungals .
Properties
IUPAC Name |
17,23-dihydroxy-3,4,6,8,12,14,20,22-octamethyl-26-oxapentacyclo[22.2.1.01,6.013,22.016,21]heptacosa-4,7,11,14,23-pentaene-25,27-dione | |
---|---|---|
Source | PubChem | |
URL | https://pubchem.ncbi.nlm.nih.gov | |
Description | Data deposited in or computed by PubChem | |
InChI |
InChI=1S/C34H46O5/c1-18-10-9-11-19(2)27-21(4)14-24-25(35)13-12-20(3)28(24)33(27,8)29(36)26-30(37)34(39-31(26)38)17-23(6)22(5)16-32(34,7)15-18/h11,14-16,20,23-25,27-28,35-36H,9-10,12-13,17H2,1-8H3 | |
Source | PubChem | |
URL | https://pubchem.ncbi.nlm.nih.gov | |
Description | Data deposited in or computed by PubChem | |
InChI Key |
DACFQDZSKBTCCU-UHFFFAOYSA-N | |
Source | PubChem | |
URL | https://pubchem.ncbi.nlm.nih.gov | |
Description | Data deposited in or computed by PubChem | |
Canonical SMILES |
CC1CCC(C2C1C3(C(C(=CCCC(=CC4(C=C(C(CC45C(=O)C(=C3O)C(=O)O5)C)C)C)C)C)C(=C2)C)C)O | |
Source | PubChem | |
URL | https://pubchem.ncbi.nlm.nih.gov | |
Description | Data deposited in or computed by PubChem | |
Molecular Formula |
C34H46O5 | |
Source | PubChem | |
URL | https://pubchem.ncbi.nlm.nih.gov | |
Description | Data deposited in or computed by PubChem | |
Molecular Weight |
534.7 g/mol | |
Source | PubChem | |
URL | https://pubchem.ncbi.nlm.nih.gov | |
Description | Data deposited in or computed by PubChem | |
Retrosynthesis Analysis
AI-Powered Synthesis Planning: Our tool employs the Template_relevance Pistachio, Template_relevance Bkms_metabolic, Template_relevance Pistachio_ringbreaker, Template_relevance Reaxys, Template_relevance Reaxys_biocatalysis model, leveraging a vast database of chemical reactions to predict feasible synthetic routes.
One-Step Synthesis Focus: Specifically designed for one-step synthesis, it provides concise and direct routes for your target compounds, streamlining the synthesis process.
Accurate Predictions: Utilizing the extensive PISTACHIO, BKMS_METABOLIC, PISTACHIO_RINGBREAKER, REAXYS, REAXYS_BIOCATALYSIS database, our tool offers high-accuracy predictions, reflecting the latest in chemical research and data.
Strategy Settings
Precursor scoring | Relevance Heuristic |
---|---|
Min. plausibility | 0.01 |
Model | Template_relevance |
Template Set | Pistachio/Bkms_metabolic/Pistachio_ringbreaker/Reaxys/Reaxys_biocatalysis |
Top-N result to add to graph | 6 |
Feasible Synthetic Routes
Q1: What are the potential therapeutic targets of Tetromycin B and how does it interact with them?
A1: Research indicates that this compound displays time-dependent inhibition of cathepsin L-like proteases []. While the exact mechanism of action is still under investigation, this suggests that this compound might bind to and inhibit the activity of these proteases. Cathepsin L-like proteases play crucial roles in various physiological processes, and their dysregulation is implicated in diseases like parasitic infections and cancer. By inhibiting these proteases, this compound could potentially interfere with the life cycle of parasites like Trypanosoma brucei or modulate disease progression.
Q2: What is known about the antiparasitic activity of this compound?
A2: this compound has shown promising in vitro activity against Trypanosoma brucei [], the parasite responsible for African trypanosomiasis (sleeping sickness) in humans. Further research is needed to determine its efficacy in animal models and to elucidate the underlying mechanisms of its antiparasitic action.
Disclaimer and Information on In-Vitro Research Products
Please be aware that all articles and product information presented on BenchChem are intended solely for informational purposes. The products available for purchase on BenchChem are specifically designed for in-vitro studies, which are conducted outside of living organisms. In-vitro studies, derived from the Latin term "in glass," involve experiments performed in controlled laboratory settings using cells or tissues. It is important to note that these products are not categorized as medicines or drugs, and they have not received approval from the FDA for the prevention, treatment, or cure of any medical condition, ailment, or disease. We must emphasize that any form of bodily introduction of these products into humans or animals is strictly prohibited by law. It is essential to adhere to these guidelines to ensure compliance with legal and ethical standards in research and experimentation.