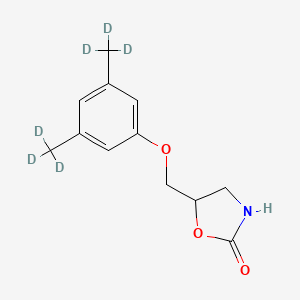
Metaxalone-d6
- Click on QUICK INQUIRY to receive a quote from our team of experts.
- With the quality product at a COMPETITIVE price, you can focus more on your research.
Overview
Description
Metaxalone-d6 is intended for use as an internal standard for the quantification of metaxalone by GC-or LC-MS. Metaxalone is a skeletal muscle relaxant. It inhibits the proliferation of, and induces apoptosis in, RAW 264.7 cells in vitro when used at concentrations ranging from 1 to 100 µM. Metaxalone also reduces LPS-induced increases in COX-1, COX-2, and NF-kB levels and inhibits LPS-induced production of TNF-α, IL-6, and prostaglandin E2 (PGE2; ) in RAW 264.7 cells. Formulations containing metaxalone have been used in the treatment of lower back pain.
Mechanism of Action
Target of Action
Metaxalone-d6, a deuterium-labeled version of Metaxalone , primarily targets the central nervous system (CNS) . It is used as a muscle relaxant , and its main mechanism of action is thought to involve general CNS depression .
Mode of Action
This means that this compound likely works by slowing down brain activity, allowing the muscles to relax and reducing pain .
Biochemical Pathways
While the specific biochemical pathways affected by this compound are not fully known, it has been observed that Metaxalone can inhibit the proliferation of, and induce apoptosis in, RAW 264.7 cells in vitro when used at concentrations ranging from 1 to 100 µM . Metaxalone also reduces LPS-induced increases in COX-1, COX-2, and NF-kB levels and inhibits LPS-induced production of TNF-α, IL-6, and prostaglandin E2 (PGE2) in RAW 264.7 cells .
Pharmacokinetics
The pharmacokinetics of Metaxalone, the parent compound of this compound, have been studied in healthy adult volunteers . The peak plasma concentrations of Metaxalone occur approximately 3 hours after a 400 mg oral dose under fasted conditions . Thereafter, Metaxalone concentrations decline log-linearly with a terminal half-life of 9.0 ± 4.8 hours . Doubling the dose of Metaxalone from 400 mg to 800 mg results in a roughly proportional increase in Metaxalone exposure .
Result of Action
Instead, its effects are likely due to its ability to depress the CNS, which can help to alleviate muscle spasms .
Action Environment
It is known that the bioavailability of metaxalone, the parent compound of this compound, is increased when taken with food . This suggests that factors such as diet could potentially influence the action of this compound.
Biochemical Analysis
Biochemical Properties
Metaxalone-d6 plays a significant role in biochemical reactions due to its interaction with various enzymes and proteins. It is known to inhibit the proliferation of RAW 264.7 cells and induce apoptosis at concentrations ranging from 1 to 100 µM . This compound also reduces lipopolysaccharide (LPS)-induced increases in cyclooxygenase-1 (COX-1), cyclooxygenase-2 (COX-2), and nuclear factor kappa-light-chain-enhancer of activated B cells (NF-κB) levels. Additionally, it inhibits the production of tumor necrosis factor-alpha (TNF-α), interleukin-6 (IL-6), and prostaglandin E2 (PGE2) in these cells .
Cellular Effects
This compound exerts various effects on different cell types and cellular processes. It influences cell function by modulating cell signaling pathways, gene expression, and cellular metabolism. For instance, this compound has been shown to suppress the production of inflammatory cytokines in mouse macrophages, thereby reducing inflammation . This compound also affects the central nervous system by inducing general depression, which contributes to its muscle relaxant properties .
Molecular Mechanism
The molecular mechanism of action of this compound involves several key interactions at the molecular level. It binds to specific biomolecules, leading to enzyme inhibition or activation and changes in gene expression. This compound inhibits the activity of COX-1 and COX-2 enzymes, which are involved in the inflammatory response . Additionally, it modulates the NF-κB signaling pathway, which plays a crucial role in regulating immune response and inflammation .
Temporal Effects in Laboratory Settings
In laboratory settings, the effects of this compound change over time. The compound is relatively stable, with a half-life of approximately 9.2 hours . Over extended periods, this compound maintains its efficacy in reducing inflammation and muscle spasms.
Dosage Effects in Animal Models
The effects of this compound vary with different dosages in animal models. At lower doses, it effectively reduces muscle spasms and inflammation without causing significant adverse effects . At higher doses, this compound may induce toxic effects, including central nervous system depression and potential liver toxicity . It is crucial to determine the optimal dosage to balance efficacy and safety in animal studies.
Metabolic Pathways
This compound is involved in various metabolic pathways, primarily in the liver. It is metabolized by hepatic enzymes and excreted in the urine as unidentified metabolites . The metabolic process involves interactions with enzymes such as cytochrome P450, which play a role in the biotransformation of the compound . Understanding these metabolic pathways is essential for optimizing the use of this compound in therapeutic applications.
Transport and Distribution
Within cells and tissues, this compound is transported and distributed through specific transporters and binding proteins. The compound’s distribution is influenced by its interactions with plasma proteins, which facilitate its transport to target tissues . This compound accumulates in muscle tissues, where it exerts its muscle relaxant effects . Its transport and distribution are critical for achieving the desired therapeutic outcomes.
Subcellular Localization
This compound exhibits specific subcellular localization, which affects its activity and function. The compound is primarily localized in the cytoplasm and mitochondria of cells . This localization is facilitated by targeting signals and post-translational modifications that direct this compound to these compartments. The subcellular distribution of this compound is crucial for its role in modulating cellular processes and achieving its therapeutic effects.
Biological Activity
Metaxalone-d6 is a deuterated form of metaxalone, a muscle relaxant primarily used for the symptomatic treatment of musculoskeletal pain. The biological activity of this compound is significant for pharmacokinetic studies, particularly in understanding the drug's metabolism and effects within the human body.
Overview of Metaxalone
Metaxalone (chemical formula: C12H15NO3) functions as an interneuronal blocking agent that acts on the central nervous system (CNS) to produce muscle relaxant effects. It is commonly prescribed for conditions such as strains and sprains, where muscle relaxation is necessary to alleviate pain and discomfort .
Pharmacokinetics of this compound
The pharmacokinetic properties of this compound are crucial for evaluating its biological activity. A study demonstrated that after administering a 400 mg dose of metaxalone, peak plasma concentration (Cmax) was reached approximately 3 hours post-dose under fasting conditions. Increasing the dose to 800 mg resulted in a proportional increase in drug concentration, indicating a linear pharmacokinetic profile .
Key Pharmacokinetic Parameters
Parameter | Value (400 mg Dose) | Value (800 mg Dose) |
---|---|---|
Cmax | ~3 hours | Increased |
Tmax | ~3 hours | Delayed by 1 hour |
AUC | Increased by 123.5% | Increased |
Half-life | Decreased by 6.6 h | Not specified |
This compound, like its parent compound, exhibits muscle relaxant properties by acting on the CNS. It is suggested that it modulates neurotransmitter release and inhibits neuronal excitability, leading to muscle relaxation . The deuterium labeling in this compound allows for improved tracking in metabolic studies, providing insights into its bioavailability and metabolic pathways.
Case Studies on Biological Activity
- Bioavailability Study : A clinical trial involving eleven participants assessed the bioavailability of a newly developed metaxalone formulation compared to an innovator drug. The study was designed as a two-period crossover trial under fed conditions, which showed that the new formulation was well-tolerated and exhibited comparable pharmacokinetic parameters to the reference product .
- Adverse Reactions : Common adverse reactions noted during trials include lethargy, dizziness, nausea, and gastrointestinal disturbances. Monitoring vital signs during these studies indicated that serious adverse events were minimal and manageable .
Properties
IUPAC Name |
5-[[3,5-bis(trideuteriomethyl)phenoxy]methyl]-1,3-oxazolidin-2-one |
Source
|
---|---|---|
Source | PubChem | |
URL | https://pubchem.ncbi.nlm.nih.gov | |
Description | Data deposited in or computed by PubChem | |
InChI |
InChI=1S/C12H15NO3/c1-8-3-9(2)5-10(4-8)15-7-11-6-13-12(14)16-11/h3-5,11H,6-7H2,1-2H3,(H,13,14)/i1D3,2D3 |
Source
|
Source | PubChem | |
URL | https://pubchem.ncbi.nlm.nih.gov | |
Description | Data deposited in or computed by PubChem | |
InChI Key |
IMWZZHHPURKASS-WFGJKAKNSA-N |
Source
|
Source | PubChem | |
URL | https://pubchem.ncbi.nlm.nih.gov | |
Description | Data deposited in or computed by PubChem | |
Canonical SMILES |
CC1=CC(=CC(=C1)OCC2CNC(=O)O2)C |
Source
|
Source | PubChem | |
URL | https://pubchem.ncbi.nlm.nih.gov | |
Description | Data deposited in or computed by PubChem | |
Isomeric SMILES |
[2H]C([2H])([2H])C1=CC(=CC(=C1)OCC2CNC(=O)O2)C([2H])([2H])[2H] |
Source
|
Source | PubChem | |
URL | https://pubchem.ncbi.nlm.nih.gov | |
Description | Data deposited in or computed by PubChem | |
Molecular Formula |
C12H15NO3 |
Source
|
Source | PubChem | |
URL | https://pubchem.ncbi.nlm.nih.gov | |
Description | Data deposited in or computed by PubChem | |
Molecular Weight |
227.29 g/mol |
Source
|
Source | PubChem | |
URL | https://pubchem.ncbi.nlm.nih.gov | |
Description | Data deposited in or computed by PubChem | |
Q1: What is the significance of using Metaxalone-d6 as an internal standard in the quantification of Metaxalone?
A: this compound, a deuterated form of Metaxalone, shares nearly identical chemical properties with the analyte (Metaxalone) except for a slight mass difference due to the presence of six deuterium atoms. This makes it an ideal internal standard for LC-MS/MS analysis. When added to the plasma samples, this compound behaves similarly to Metaxalone during sample preparation and chromatographic separation. By comparing the signal ratios of Metaxalone and this compound, the method compensates for potential variations during these steps, leading to more accurate and reliable quantification of Metaxalone in human plasma [].
Disclaimer and Information on In-Vitro Research Products
Please be aware that all articles and product information presented on BenchChem are intended solely for informational purposes. The products available for purchase on BenchChem are specifically designed for in-vitro studies, which are conducted outside of living organisms. In-vitro studies, derived from the Latin term "in glass," involve experiments performed in controlled laboratory settings using cells or tissues. It is important to note that these products are not categorized as medicines or drugs, and they have not received approval from the FDA for the prevention, treatment, or cure of any medical condition, ailment, or disease. We must emphasize that any form of bodily introduction of these products into humans or animals is strictly prohibited by law. It is essential to adhere to these guidelines to ensure compliance with legal and ethical standards in research and experimentation.