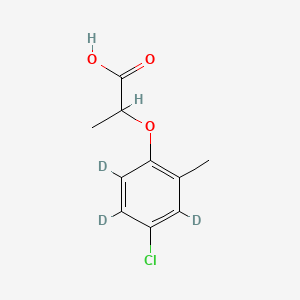
Mecoprop-d3
Overview
Description
Mecoprop-d3, also known as 2-(4-chloro-2-methylphenoxy)-2,3,5-d3-propanoic acid, is a deuterium-labeled analog of Mecoprop. It is primarily used as a reference standard in environmental testing and research. The compound is a stable isotope-labeled herbicide, which allows for precise quantification and analysis in various scientific studies .
Mechanism of Action
Target of Action
Mecoprop-d3, a deuterium-labeled variant of Mecoprop, is primarily used to control broadleaf weeds . Its primary targets are these broadleaf weeds, including prostate chickweed, stitchwort, ground ivy, knotweed, clover, and plantain .
Mode of Action
This compound works by mimicking the plant hormone Indole-3-acetic acid (IAA), also known as auxin . This mimicry leads to uncontrolled growth in the target weeds, disrupting their normal cell division . The uncontrolled growth eventually leads to the death of the weed.
Biochemical Pathways
It is known that the compound interferes with the normal functioning of auxin, a key hormone involved in plant growth and development . This interference disrupts normal cell division and causes uncontrolled growth, which is detrimental to the plant’s survival .
Pharmacokinetics
It is known that mecoprop, the non-deuterated form of the compound, readily leaches in soil but also quickly biodegrades
Result of Action
The primary result of this compound’s action is the death of broadleaf weeds. By causing uncontrolled growth and disrupting normal cell division, this compound effectively eliminates these weeds from the environment .
Action Environment
The action, efficacy, and stability of this compound can be influenced by various environmental factors. For instance, Mecoprop readily biodegrades in soil , suggesting that soil composition and microbial activity could impact the persistence and effectiveness of this compound. Additionally, exposure to this compound would be primarily occupational, through dermal contact with the herbicide and treated surfaces, and inhalation and ingestion of spray droplets .
Biochemical Analysis
Biochemical Properties
The biochemical properties of Mecoprop-d3 are expected to be similar to those of Mecoprop, given their structural similarity. Mecoprop is known to mimic the plant hormone IAA (auxin), causing uncontrolled growth in broadleaf weeds, leading to their death
Cellular Effects
As a derivative of Mecoprop, it may influence cell function by disrupting normal cell division . This disruption is what leads to the death of broadleaf weeds when they are exposed to Mecoprop .
Molecular Mechanism
Mecoprop, the parent compound, is known to mimic the plant hormone IAA (auxin), leading to uncontrolled growth in broadleaf weeds . It is likely that this compound shares a similar mechanism of action.
Temporal Effects in Laboratory Settings
There is limited information available on the temporal effects of this compound in laboratory settings. Mecoprop, the parent compound, is known to readily biodegrade in soil, with reported half-lives generally ranging from 3 to 21 days . It is possible that this compound exhibits similar temporal characteristics.
Metabolic Pathways
Mecoprop, the parent compound, is known to be metabolized by various enzymes in plants .
Transport and Distribution
Mecoprop, the parent compound, is known to be very mobile in soil and has been detected in groundwater samples .
Preparation Methods
Synthetic Routes and Reaction Conditions: The synthesis of Mecoprop-d3 involves the incorporation of deuterium atoms into the Mecoprop molecule. This can be achieved through various methods, including catalytic hydrogen-deuterium exchange reactions. The reaction typically involves the use of deuterium gas (D2) in the presence of a suitable catalyst under controlled conditions to replace hydrogen atoms with deuterium .
Industrial Production Methods: Industrial production of this compound follows similar synthetic routes but on a larger scale. The process involves the use of high-purity deuterium gas and advanced catalytic systems to ensure efficient and consistent incorporation of deuterium atoms. The final product is then purified and characterized to meet stringent quality standards for use as a reference material .
Chemical Reactions Analysis
Types of Reactions: Mecoprop-d3 undergoes various chemical reactions, including:
Oxidation: this compound can be oxidized to form corresponding carboxylic acids and other oxidation products.
Reduction: Reduction reactions can convert this compound to its corresponding alcohols or other reduced forms.
Substitution: this compound can undergo nucleophilic substitution reactions, where the chlorine atom is replaced by other nucleophiles.
Common Reagents and Conditions:
Oxidation: Common oxidizing agents include potassium permanganate (KMnO4) and chromium trioxide (CrO3) under acidic conditions.
Reduction: Reducing agents such as lithium aluminum hydride (LiAlH4) or sodium borohydride (NaBH4) are commonly used.
Substitution: Nucleophiles like sodium hydroxide (NaOH) or potassium hydroxide (KOH) in aqueous or alcoholic solutions are used for substitution reactions.
Major Products Formed:
Oxidation: Formation of carboxylic acids and other oxidized derivatives.
Reduction: Formation of alcohols and other reduced derivatives.
Substitution: Formation of substituted phenoxypropanoic acids with different functional groups.
Scientific Research Applications
Mecoprop-d3 is widely used in scientific research due to its stable isotope labeling. Some key applications include:
Environmental Testing: Used as a reference standard for the quantification of Mecoprop residues in environmental samples, such as soil and water.
Pharmacokinetic Studies: Employed in drug metabolism and pharmacokinetic studies to trace the distribution and elimination of Mecoprop in biological systems.
Analytical Chemistry: Utilized in the development and validation of analytical methods, including chromatography and mass spectrometry, for the detection and quantification of herbicides.
Toxicology Studies: Used to study the toxicological effects and metabolic pathways of Mecoprop in various organisms
Comparison with Similar Compounds
Mecoprop: The non-deuterated form of Mecoprop-d3, widely used as a herbicide.
2,4-Dichlorophenoxyacetic Acid (2,4-D): Another phenoxy herbicide with similar applications and mode of action.
Dicamba: A benzoic acid herbicide used for controlling broadleaf weeds.
Uniqueness of this compound: this compound’s uniqueness lies in its deuterium labeling, which provides distinct advantages in research applications. The stable isotope labeling allows for precise quantification and tracking in various analytical and pharmacokinetic studies. This makes this compound an invaluable tool in environmental testing, toxicology, and analytical chemistry .
Biological Activity
Mecoprop-d3, a deuterated derivative of mecoprop, is a herbicide primarily used for controlling broadleaf weeds. Understanding its biological activity is crucial for assessing its safety and efficacy in agricultural settings. This article synthesizes findings from various studies, focusing on its pharmacokinetics, toxicological effects, and environmental impact.
Pharmacokinetics
Table 1: Pharmacokinetic Parameters of this compound
Parameter | Male Rats | Female Rats |
---|---|---|
Bioavailability | 90-100% | 80-95% |
Cmax (µg/g) | 27.8 | 31.5 |
Tmax (hr) | 1.8 | 2.7 |
T1/2 (hr) | 6.4 | 4.2 |
Metabolism
Acute Toxicity
This compound has been evaluated for its acute toxicity through various studies:
- Oral LD50 : Approximately 431 mg/kg body weight in rats.
- Dermal LD50 : Greater than 2000 mg/kg body weight.
- Inhalation LC50 : More than 2.13 mg/L over a four-hour exposure period .
These findings suggest that while mecocprop-d3 poses some risk through ingestion or inhalation, it is relatively safe upon dermal exposure.
Long-term Toxicity
Ecotoxicological Impact
This compound's environmental persistence and potential for bioaccumulation necessitate careful consideration in agricultural applications. Studies indicate that while it can degrade in soil and water environments, its metabolites may pose risks to non-target organisms . The degradation pathways are influenced by microbial activity and environmental conditions, which can vary widely across different ecosystems.
Table 2: Ecotoxicological Studies Summary
Case Study: Worker Exposure Risk Assessment
Case Study: Environmental Monitoring
Monitoring programs have been initiated to evaluate the presence of mecocprop-d3 and its metabolites in groundwater and surface water sources near agricultural sites. Preliminary results indicate variable levels of contamination, prompting further investigation into the long-term ecological impacts of herbicide use in these areas .
Properties
IUPAC Name |
2-(4-chloro-2,3,5-trideuterio-6-methylphenoxy)propanoic acid | |
---|---|---|
Source | PubChem | |
URL | https://pubchem.ncbi.nlm.nih.gov | |
Description | Data deposited in or computed by PubChem | |
InChI |
InChI=1S/C10H11ClO3/c1-6-5-8(11)3-4-9(6)14-7(2)10(12)13/h3-5,7H,1-2H3,(H,12,13)/i3D,4D,5D | |
Source | PubChem | |
URL | https://pubchem.ncbi.nlm.nih.gov | |
Description | Data deposited in or computed by PubChem | |
InChI Key |
WNTGYJSOUMFZEP-QGZYMEECSA-N | |
Source | PubChem | |
URL | https://pubchem.ncbi.nlm.nih.gov | |
Description | Data deposited in or computed by PubChem | |
Canonical SMILES |
CC1=C(C=CC(=C1)Cl)OC(C)C(=O)O | |
Source | PubChem | |
URL | https://pubchem.ncbi.nlm.nih.gov | |
Description | Data deposited in or computed by PubChem | |
Isomeric SMILES |
[2H]C1=C(C(=C(C(=C1OC(C)C(=O)O)C)[2H])Cl)[2H] | |
Source | PubChem | |
URL | https://pubchem.ncbi.nlm.nih.gov | |
Description | Data deposited in or computed by PubChem | |
Molecular Formula |
C10H11ClO3 | |
Source | PubChem | |
URL | https://pubchem.ncbi.nlm.nih.gov | |
Description | Data deposited in or computed by PubChem | |
DSSTOX Substance ID |
DTXSID20662063 | |
Record name | 2-{[4-Chloro-2-methyl(~2~H_3_)phenyl]oxy}propanoic acid | |
Source | EPA DSSTox | |
URL | https://comptox.epa.gov/dashboard/DTXSID20662063 | |
Description | DSSTox provides a high quality public chemistry resource for supporting improved predictive toxicology. | |
Molecular Weight |
217.66 g/mol | |
Source | PubChem | |
URL | https://pubchem.ncbi.nlm.nih.gov | |
Description | Data deposited in or computed by PubChem | |
CAS No. |
352431-15-3 | |
Record name | 2-{[4-Chloro-2-methyl(~2~H_3_)phenyl]oxy}propanoic acid | |
Source | EPA DSSTox | |
URL | https://comptox.epa.gov/dashboard/DTXSID20662063 | |
Description | DSSTox provides a high quality public chemistry resource for supporting improved predictive toxicology. | |
Disclaimer and Information on In-Vitro Research Products
Please be aware that all articles and product information presented on BenchChem are intended solely for informational purposes. The products available for purchase on BenchChem are specifically designed for in-vitro studies, which are conducted outside of living organisms. In-vitro studies, derived from the Latin term "in glass," involve experiments performed in controlled laboratory settings using cells or tissues. It is important to note that these products are not categorized as medicines or drugs, and they have not received approval from the FDA for the prevention, treatment, or cure of any medical condition, ailment, or disease. We must emphasize that any form of bodily introduction of these products into humans or animals is strictly prohibited by law. It is essential to adhere to these guidelines to ensure compliance with legal and ethical standards in research and experimentation.