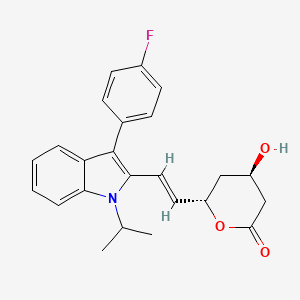
Fluvastatin Lactone
Overview
Description
Fluvastatin Lactone is a derivative of Fluvastatin, a synthetic statin used to lower lipid levels and reduce the risk of cardiovascular diseases such as myocardial infarction and stroke . Fluvastatin is an HMG-CoA reductase inhibitor, which plays a crucial role in cholesterol biosynthesis by catalyzing the conversion of HMG-CoA to mevalonate . The lactone form of Fluvastatin is known for its stability and unique pharmacological properties .
Mechanism of Action
Target of Action
Fluvastatin Lactone primarily targets the enzyme hydroxymethylglutaryl-coenzyme A (HMG-CoA) reductase . This enzyme plays a crucial role in cholesterol biosynthesis, catalyzing the conversion of HMG-CoA to mevalonic acid, which is the rate-limiting step in the process .
Mode of Action
This compound acts as a competitive inhibitor of HMG-CoA reductase . By binding to the enzyme, it prevents the conversion of HMG-CoA to mevalonic acid, thereby inhibiting cholesterol synthesis . This results in a decrease in intracellular cholesterol levels, which triggers an increase in the number of low-density lipoprotein (LDL) receptors on the cell surface. These receptors bind to circulating LDL, leading to its removal from the bloodstream and a reduction in blood cholesterol levels .
Biochemical Pathways
The primary biochemical pathway affected by this compound is the mevalonate pathway . By inhibiting HMG-CoA reductase, this compound reduces the production of mevalonate, a key intermediate in the synthesis of cholesterol and other isoprenoids . These isoprenoids are vital for various cellular functions, ranging from cholesterol synthesis to the control of cell growth and differentiation .
Pharmacokinetics
This compound is quickly and almost completely absorbed from the gut . Due to its first-pass effect, its bioavailability is lower, about 24–30% . Over 98% of the substance is bound to plasma proteins . After absorption, it is nearly completely extracted and metabolized in the liver to 2 hydroxylated metabolites and an N-desisopropyl metabolite, which are excreted in the bile . Approximately 95% of a dose is recovered in the feces, with 60% of a dose recovered as the 3 metabolites .
Result of Action
The molecular and cellular effects of this compound’s action primarily involve a reduction in cholesterol biosynthesis, leading to decreased intracellular cholesterol levels . This triggers an increase in the number of LDL receptors on the cell surface, leading to enhanced uptake and clearance of LDL cholesterol from the bloodstream . As a result, this compound effectively reduces plasma cholesterol levels and prevents cardiovascular disease .
Action Environment
The action of this compound can be influenced by various environmental factors. The drug’s interaction with other drugs and food supplements is associated with altered bioavailability . Moreover, certain drugs known to cause drug-drug interactions with statins show that reduction potential and cellular uptake properties are useful predictors of such interactions .
Biochemical Analysis
Biochemical Properties
Fluvastatin Lactone interacts with the enzyme HMG-CoA reductase, which catalyzes the conversion of HMG-CoA to mevalonic acid . This is the rate-limiting step in cholesterol biosynthesis . By inhibiting this enzyme, this compound effectively reduces the production of cholesterol in the body .
Cellular Effects
This compound has a significant impact on various types of cells and cellular processes. It influences cell function by altering cell signaling pathways, gene expression, and cellular metabolism . Specifically, it reduces the synthesis of cholesterol, a critical component of cell membranes, and modulates the function of proteins involved in lipid metabolism .
Molecular Mechanism
The mechanism of action of this compound involves its binding to HMG-CoA reductase, inhibiting the enzyme’s activity . This prevents the conversion of HMG-CoA to mevalonic acid, thereby reducing the production of cholesterol . The inhibition of this enzyme also leads to changes in gene expression related to cholesterol biosynthesis .
Temporal Effects in Laboratory Settings
Over time, the effects of this compound can change in laboratory settings. For instance, the drug’s stability, degradation, and long-term effects on cellular function have been observed in in vitro or in vivo studies . The lactone forms of statins, including this compound, play a major role in the transport of statins across the cell membrane, and in the metabolism and clearance from the body .
Dosage Effects in Animal Models
The effects of this compound can vary with different dosages in animal models . For instance, at certain threshold levels, the drug can effectively reduce cholesterol levels. At high doses, toxic or adverse effects may be observed .
Metabolic Pathways
This compound is involved in the cholesterol biosynthesis pathway . It interacts with the enzyme HMG-CoA reductase and inhibits its activity . This leads to a decrease in the production of mevalonic acid, a key intermediate in the biosynthesis of cholesterol .
Transport and Distribution
This compound is transported and distributed within cells and tissues . It is preferentially transported across the cell membrane and is then metabolized and cleared from the body . The preferred cellular uptake of this compound has implications for its distribution within the body and its accumulation in certain tissues .
Subcellular Localization
This compound, like other statins, is primarily localized in the liver, where it exerts its effects . The liver is the primary site of cholesterol synthesis, and hence, the main target of this compound. The drug’s activity in the liver leads to a reduction in cholesterol levels, thereby contributing to its therapeutic effects .
Preparation Methods
Synthetic Routes and Reaction Conditions
Fluvastatin Lactone can be synthesized through various chemical routes. One common method involves the cyclization of Fluvastatin under acidic conditions to form the lactone ring . The reaction typically requires a non-polar stationary phase and an aqueous phase buffer like tetramethylammonium hydroxide with a pH of 7.2 for the elution of the parent component and its respective degradants .
Industrial Production Methods
Industrial production of this compound involves large-scale chemical synthesis using optimized reaction conditions to ensure high yield and purity. The process often includes steps such as esterification, hydrolysis, and lactonization under controlled conditions to produce the desired lactone form .
Chemical Reactions Analysis
Types of Reactions
Fluvastatin Lactone undergoes various chemical reactions, including:
Oxidation: The compound is susceptible to oxidative degradation, leading to the formation of epoxide impurities.
Hydrolysis: It can hydrolyze back to its hydroxy acid form under basic conditions.
Lactonization: The conversion of the hydroxy acid form to the lactone form under acidic conditions.
Common Reagents and Conditions
Oxidation: Common oxidizing agents include hydrogen peroxide and molecular oxygen.
Hydrolysis: Basic conditions using sodium hydroxide or potassium hydroxide.
Lactonization: Acidic conditions using hydrochloric acid or sulfuric acid.
Major Products Formed
Oxidation: Epoxide impurities.
Hydrolysis: Hydroxy acid form of Fluvastatin.
Lactonization: this compound.
Scientific Research Applications
Fluvastatin Lactone has a wide range of scientific research applications, including:
Comparison with Similar Compounds
Fluvastatin Lactone is unique among statins due to its entirely synthetic origin and distinct biopharmaceutic profile . Similar compounds include:
Simvastatin: A lactone prodrug that is hydrolyzed to its active hydroxy acid form.
Lovastatin: Another lactone prodrug with similar pharmacological properties.
Atorvastatin: A synthetic statin that exists in its active hydroxy acid form.
Pravastatin: A statin derived from fungal metabolites, known for its water solubility and distinct pharmacokinetic profile.
This compound’s uniqueness lies in its synthetic origin, stability, and favorable safety profile, making it a highly attractive option for lipid-lowering therapy .
Properties
IUPAC Name |
(4R,6S)-6-[(E)-2-[3-(4-fluorophenyl)-1-propan-2-ylindol-2-yl]ethenyl]-4-hydroxyoxan-2-one | |
---|---|---|
Source | PubChem | |
URL | https://pubchem.ncbi.nlm.nih.gov | |
Description | Data deposited in or computed by PubChem | |
InChI |
InChI=1S/C24H24FNO3/c1-15(2)26-21-6-4-3-5-20(21)24(16-7-9-17(25)10-8-16)22(26)12-11-19-13-18(27)14-23(28)29-19/h3-12,15,18-19,27H,13-14H2,1-2H3/b12-11+/t18-,19-/m1/s1 | |
Source | PubChem | |
URL | https://pubchem.ncbi.nlm.nih.gov | |
Description | Data deposited in or computed by PubChem | |
InChI Key |
VPXDEUFAXJFWBG-MCBHFWOFSA-N | |
Source | PubChem | |
URL | https://pubchem.ncbi.nlm.nih.gov | |
Description | Data deposited in or computed by PubChem | |
Canonical SMILES |
CC(C)N1C2=CC=CC=C2C(=C1C=CC3CC(CC(=O)O3)O)C4=CC=C(C=C4)F | |
Source | PubChem | |
URL | https://pubchem.ncbi.nlm.nih.gov | |
Description | Data deposited in or computed by PubChem | |
Isomeric SMILES |
CC(C)N1C2=CC=CC=C2C(=C1/C=C/[C@@H]3C[C@H](CC(=O)O3)O)C4=CC=C(C=C4)F | |
Source | PubChem | |
URL | https://pubchem.ncbi.nlm.nih.gov | |
Description | Data deposited in or computed by PubChem | |
Molecular Formula |
C24H24FNO3 | |
Source | PubChem | |
URL | https://pubchem.ncbi.nlm.nih.gov | |
Description | Data deposited in or computed by PubChem | |
DSSTOX Substance ID |
DTXSID50652593 | |
Record name | (4R,6S)-6-{(E)-2-[3-(4-Fluorophenyl)-1-(propan-2-yl)-1H-indol-2-yl]ethenyl}-4-hydroxyoxan-2-one | |
Source | EPA DSSTox | |
URL | https://comptox.epa.gov/dashboard/DTXSID50652593 | |
Description | DSSTox provides a high quality public chemistry resource for supporting improved predictive toxicology. | |
Molecular Weight |
393.4 g/mol | |
Source | PubChem | |
URL | https://pubchem.ncbi.nlm.nih.gov | |
Description | Data deposited in or computed by PubChem | |
CAS No. |
94061-83-3 | |
Record name | (4R,6S)-6-[(1E)-2-[3-(4-Fluorophenyl)-1-(1-methylethyl)-1H-indol-2-yl]ethenyl]tetrahydro-4-hydroxy-2H-pyran-2-one | |
Source | CAS Common Chemistry | |
URL | https://commonchemistry.cas.org/detail?cas_rn=94061-83-3 | |
Description | CAS Common Chemistry is an open community resource for accessing chemical information. Nearly 500,000 chemical substances from CAS REGISTRY cover areas of community interest, including common and frequently regulated chemicals, and those relevant to high school and undergraduate chemistry classes. This chemical information, curated by our expert scientists, is provided in alignment with our mission as a division of the American Chemical Society. | |
Explanation | The data from CAS Common Chemistry is provided under a CC-BY-NC 4.0 license, unless otherwise stated. | |
Record name | (4R,6S)-6-{(E)-2-[3-(4-Fluorophenyl)-1-(propan-2-yl)-1H-indol-2-yl]ethenyl}-4-hydroxyoxan-2-one | |
Source | EPA DSSTox | |
URL | https://comptox.epa.gov/dashboard/DTXSID50652593 | |
Description | DSSTox provides a high quality public chemistry resource for supporting improved predictive toxicology. | |
Disclaimer and Information on In-Vitro Research Products
Please be aware that all articles and product information presented on BenchChem are intended solely for informational purposes. The products available for purchase on BenchChem are specifically designed for in-vitro studies, which are conducted outside of living organisms. In-vitro studies, derived from the Latin term "in glass," involve experiments performed in controlled laboratory settings using cells or tissues. It is important to note that these products are not categorized as medicines or drugs, and they have not received approval from the FDA for the prevention, treatment, or cure of any medical condition, ailment, or disease. We must emphasize that any form of bodily introduction of these products into humans or animals is strictly prohibited by law. It is essential to adhere to these guidelines to ensure compliance with legal and ethical standards in research and experimentation.