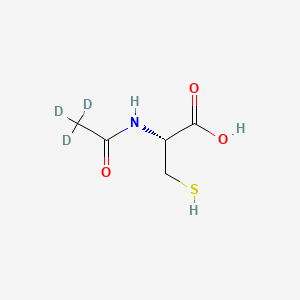
N-Acetyl-L-cysteine-d3
Overview
Description
N-Acetyl-L-cysteine-d3 is a deuterated form of N-Acetyl-L-cysteine, where three hydrogen atoms are replaced with deuterium. This compound is known for its antioxidant properties and is widely used in scientific research and medicine. It serves as a precursor to glutathione, a critical antioxidant in the human body, and has applications in various fields, including biochemistry, pharmaceuticals, and nutrition .
Mechanism of Action
Target of Action
N-Acetyl-L-cysteine-d3 (NAC), an acetylated derivative of the amino acid L-cysteine, primarily targets glutathione , a powerful antioxidant in the body . It is also known to act as a mucolytic agent , reducing the viscosity of mucus in the respiratory tract .
Mode of Action
NAC works by replenishing the body’s stores of glutathione, thereby helping to remove toxic substances . In the case of acetaminophen overdoses, a portion of the drug is metabolized by CYP2E1 to form the potentially toxic metabolite N-acetyl-p-benzoquinone imine (NAPQI). The amount of NAPQI produced in an overdose saturates and depletes glutathione stores. The free NAPQI binds to proteins in hepatocytes, leading to cellular damage . NAC can also thin and loosen mucus, making it easier to cough out .
Biochemical Pathways
NAC plays a crucial role in the glutathione redox chemistry , which is a central part of metabolic chemistry and strongly influences various therapies . It forms a disulfide bond in the presence of reduced glutathione, generating a spectroscopically detectable product . NAC also affects neoplastic growth in preventative, pre-neoplastic, and treatment stages .
Pharmacokinetics
Following both single and multiple-dose administration, the plasma concentration of NAC increases rapidly, reaching a peak at approximately 1.0 h. The maximum plasma concentration and extent of exposure are higher after multiple doses than after a single dose . The half-life is about 15.4-18.7 hours . The fraction of NAC excreted in urine in the 36 hours following administration is about 3.7-3.8% .
Result of Action
The administration of NAC results in a reduction in inflammation and enhancement in cell myelination and brain tropism . It also has antioxidant and anti-inflammatory properties , and it has been shown to affect neoplastic growth in preventative, pre-neoplastic, and treatment stages .
Action Environment
The bioavailability and action of NAC can be influenced by the environment. For instance, a study showed that NAC can permeabilize through cell membranes without active transport, and its chemical reactions with GSH can be observed within the lifetime of this hyperpolarized 13C probe . Another study demonstrated that NAC reaches the target and successfully crosses the blood-brain and intestinal barriers . The results of these studies suggest that the environment can influence the absorption, distribution, and efficacy of NAC.
Biochemical Analysis
Biochemical Properties
N-Acetyl-L-cysteine-d3 plays a significant role in biochemical reactions. It acts as a precursor for the amino acid L-cysteine, which helps the body create and use protective antioxidants . It directly modifies the activity of several proteins through its reducing activity . NAC has been found to exhibit antioxidant, anti-infective, and anti-inflammatory activity in pre-clinical and clinical reports .
Cellular Effects
This compound has various effects on different types of cells and cellular processes. It has been shown to prevent apoptosis in neuronal cells but induce apoptosis in smooth muscle cells . It also reduces inflammation and enhances cell myelination and brain tropism . NAC can support the body’s antioxidant level during infections, toxic assaults, inflammations, and stresses .
Molecular Mechanism
This compound exerts its effects at the molecular level through several mechanisms. It serves as a substrate for microsomal glutathione transferase , and it inhibits the activation of NF-kB and neurokinin A production, resulting in a reduction in interleukin-6 production . NAC also preserves mitochondrial function by inhibiting excessive mitophagy and promoting mitochondria biogenesis to prevent ROS production .
Temporal Effects in Laboratory Settings
In laboratory settings, this compound has shown changes in its effects over time. NAC treatment improves glucose metabolism as dose- and time-dependent in HFD-induced diabetic mice . Moreover, NAC treatment was able to prevent HFD-induced NAFLD, as evidenced by less hepatic triglyceride accumulation and lipid droplet formation compared with that of mice in the HFD group .
Dosage Effects in Animal Models
The effects of this compound vary with different dosages in animal models. Mice treated with a long-term low concentration (0.1 mM) of NAC had increased litter sizes at the ages of 7–10 months compared with age-matched controls without NAC treatment .
Metabolic Pathways
This compound is involved in the regulation of the glutamatergic system. As a precursor of L-cysteine, which results in glutathione biosynthesis, NAC is an amino acid that is needed to produce glutathione, a powerful antioxidant in the human body .
Transport and Distribution
This compound is transported and distributed within cells and tissues. The results show that the combination reaches the target and successfully crosses the blood–brain and intestinal barriers .
Subcellular Localization
It is known that NAC can act as a direct antioxidant, but more importantly, it provides the cysteine required for the production of glutathione, a powerful antioxidant produced in the body .
Preparation Methods
Synthetic Routes and Reaction Conditions
The synthesis of N-Acetyl-L-cysteine-d3 typically involves the acetylation of L-cysteine-d3. One common method includes the reaction of L-cysteine-d3 with acetic anhydride in the presence of a base such as sodium acetate. The reaction is carried out under controlled conditions to ensure high yield and purity .
Industrial Production Methods
Industrial production of this compound often employs a green synthesis approach. This involves the acetylation of L-cystine to form N, N’-diacetyl-L-cystine, followed by electrochemical reduction to produce this compound. This method is efficient, economical, and environmentally friendly, with high product quality and low waste emissions .
Chemical Reactions Analysis
Types of Reactions
N-Acetyl-L-cysteine-d3 undergoes various chemical reactions, including:
Oxidation: It can be oxidized to form disulfides.
Reduction: It can be reduced to free thiol groups.
Substitution: It can participate in nucleophilic substitution reactions.
Common Reagents and Conditions
Common reagents used in these reactions include oxidizing agents like hydrogen peroxide for oxidation, reducing agents like dithiothreitol for reduction, and bases like sodium hydroxide for substitution reactions. The reactions are typically carried out under mild conditions to preserve the integrity of the compound .
Major Products Formed
The major products formed from these reactions include disulfides from oxidation, free thiols from reduction, and substituted derivatives from nucleophilic substitution .
Scientific Research Applications
N-Acetyl-L-cysteine-d3 has a wide range of applications in scientific research:
Chemistry: Used as a reducing agent and a precursor in various chemical syntheses.
Biology: Studied for its role in cellular redox balance and as a precursor to glutathione.
Medicine: Investigated for its potential in treating conditions like oxidative stress, neurodegenerative diseases, and as an antidote for acetaminophen overdose.
Industry: Used in the production of pharmaceuticals and as a dietary supplement
Comparison with Similar Compounds
Similar Compounds
N-Acetyl-L-cysteine: The non-deuterated form, widely used for similar applications.
Glutathione: A direct antioxidant, but with limited bioavailability compared to N-Acetyl-L-cysteine-d3.
Vitamin D3: Often used in combination with this compound for enhanced antioxidant effects
Uniqueness
This compound is unique due to its deuterium content, which provides enhanced stability and potentially different pharmacokinetic properties compared to its non-deuterated counterpart. This makes it particularly valuable in research settings where isotopic labeling is required .
Properties
IUPAC Name |
(2R)-3-sulfanyl-2-[(2,2,2-trideuterioacetyl)amino]propanoic acid | |
---|---|---|
Source | PubChem | |
URL | https://pubchem.ncbi.nlm.nih.gov | |
Description | Data deposited in or computed by PubChem | |
InChI |
InChI=1S/C5H9NO3S/c1-3(7)6-4(2-10)5(8)9/h4,10H,2H2,1H3,(H,6,7)(H,8,9)/t4-/m0/s1/i1D3 | |
Source | PubChem | |
URL | https://pubchem.ncbi.nlm.nih.gov | |
Description | Data deposited in or computed by PubChem | |
InChI Key |
PWKSKIMOESPYIA-OSIBIXDNSA-N | |
Source | PubChem | |
URL | https://pubchem.ncbi.nlm.nih.gov | |
Description | Data deposited in or computed by PubChem | |
Canonical SMILES |
CC(=O)NC(CS)C(=O)O | |
Source | PubChem | |
URL | https://pubchem.ncbi.nlm.nih.gov | |
Description | Data deposited in or computed by PubChem | |
Isomeric SMILES |
[2H]C([2H])([2H])C(=O)N[C@@H](CS)C(=O)O | |
Source | PubChem | |
URL | https://pubchem.ncbi.nlm.nih.gov | |
Description | Data deposited in or computed by PubChem | |
Molecular Formula |
C5H9NO3S | |
Source | PubChem | |
URL | https://pubchem.ncbi.nlm.nih.gov | |
Description | Data deposited in or computed by PubChem | |
Molecular Weight |
166.22 g/mol | |
Source | PubChem | |
URL | https://pubchem.ncbi.nlm.nih.gov | |
Description | Data deposited in or computed by PubChem | |
CAS No. |
131685-11-5 | |
Record name | (2R)-3-sulfanyl-2-[(2,2,2-trideuterioacetyl)amino]propanoic acid | |
Source | European Chemicals Agency (ECHA) | |
URL | https://echa.europa.eu/information-on-chemicals | |
Description | The European Chemicals Agency (ECHA) is an agency of the European Union which is the driving force among regulatory authorities in implementing the EU's groundbreaking chemicals legislation for the benefit of human health and the environment as well as for innovation and competitiveness. | |
Explanation | Use of the information, documents and data from the ECHA website is subject to the terms and conditions of this Legal Notice, and subject to other binding limitations provided for under applicable law, the information, documents and data made available on the ECHA website may be reproduced, distributed and/or used, totally or in part, for non-commercial purposes provided that ECHA is acknowledged as the source: "Source: European Chemicals Agency, http://echa.europa.eu/". Such acknowledgement must be included in each copy of the material. ECHA permits and encourages organisations and individuals to create links to the ECHA website under the following cumulative conditions: Links can only be made to webpages that provide a link to the Legal Notice page. | |
Disclaimer and Information on In-Vitro Research Products
Please be aware that all articles and product information presented on BenchChem are intended solely for informational purposes. The products available for purchase on BenchChem are specifically designed for in-vitro studies, which are conducted outside of living organisms. In-vitro studies, derived from the Latin term "in glass," involve experiments performed in controlled laboratory settings using cells or tissues. It is important to note that these products are not categorized as medicines or drugs, and they have not received approval from the FDA for the prevention, treatment, or cure of any medical condition, ailment, or disease. We must emphasize that any form of bodily introduction of these products into humans or animals is strictly prohibited by law. It is essential to adhere to these guidelines to ensure compliance with legal and ethical standards in research and experimentation.