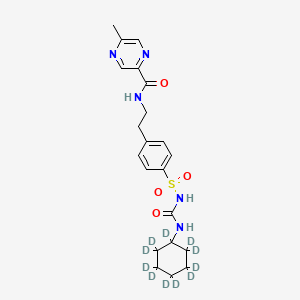
Glipizide-d11
Overview
Description
- Glipizide acts by partially blocking ATP-sensitive potassium (KATP) channels in pancreatic β cells of the islets of Langerhans .
Glipizide-d11: is a deuterium-substituted form of
Preparation Methods
- Unfortunately, specific synthetic routes and reaction conditions for Glipizide-d11 are not readily available in the literature. it is typically synthesized through deuterium incorporation during chemical synthesis.
- Industrial production methods may involve isotopic labeling techniques to introduce deuterium atoms into the Glipizide molecule.
Chemical Reactions Analysis
- Glipizide-d11 likely undergoes similar chemical reactions as Glipizide, including oxidation, reduction, and substitution reactions.
- Common reagents and conditions used in these reactions would be analogous to those employed for Glipizide.
- Major products formed from these reactions would be deuterium-labeled derivatives of Glipizide.
Scientific Research Applications
Analytical Chemistry
Internal Standard for Quantification
Glipizide-d11 is primarily utilized as an internal standard in gas chromatography (GC) and liquid chromatography-mass spectrometry (LC-MS) for the quantification of glipizide. Its deuterated nature allows for distinct peak identification and improved accuracy in mass spectrometric analysis, which is crucial for determining drug concentrations in biological samples .
Method Validation
Recent studies have demonstrated the effectiveness of using this compound in validating analytical methods for detecting glipizide alongside other antidiabetic agents such as metformin and gliclazide. The use of this compound has shown robust selectivity, specificity, and accuracy across varying concentration levels, ensuring reliable results in clinical and research settings .
Pharmacokinetics and Metabolic Studies
Mechanism of Action
Glipizide functions by stimulating insulin release from pancreatic beta cells and enhancing insulin sensitivity in peripheral tissues. Studies involving this compound have elucidated its pharmacokinetic properties, including absorption rates and metabolic pathways. For instance, it has been shown to increase insulin receptor numbers in hepatic tissues when administered in specific dosages .
Animal Models
In preclinical studies using rat models of diabetes, this compound has been effective in reducing plasma glucose and triglyceride levels while increasing plasma insulin concentrations. These findings are pivotal for understanding the drug's efficacy and safety profile in diabetic management .
Clinical Research Applications
Therapeutic Monitoring
this compound plays a significant role in therapeutic drug monitoring (TDM) for patients undergoing treatment with glipizide. By providing a reliable means to measure drug levels, clinicians can optimize dosing regimens to achieve desired glycemic control while minimizing adverse effects .
Case Studies
- A study published in the journal Oncotarget highlighted the role of glipizide (and by extension, this compound) in suppressing tumor growth through mechanisms involving angiogenesis inhibition. This suggests potential applications beyond diabetes management, indicating that glipizide may have adjunctive roles in cancer therapy .
- Another investigation focused on the combination therapy of glipizide with other agents to enhance therapeutic outcomes in type 2 diabetes patients. The results indicated improved glycemic control when glipizide was used alongside newer antidiabetic medications .
Summary Table of Applications
Application Area | Description |
---|---|
Analytical Chemistry | Internal standard for quantifying glipizide via GC/LC-MS; enhances accuracy and specificity |
Pharmacokinetics | Studies on absorption, metabolism; effects on insulin receptor dynamics |
Clinical Research | Therapeutic drug monitoring; case studies on cancer suppression and combination therapies |
Mechanism of Action
- Glipizide exerts its effects by partially blocking ATP-sensitive potassium channels (KATP) in pancreatic β cells.
- This action leads to increased insulin secretion and improved glucose utilization.
Comparison with Similar Compounds
- Glipizide-d11’s uniqueness lies in its deuterium substitution, which affects its pharmacokinetic and metabolic profiles.
- Similar compounds include other sulfonylureas like Glyburide (Glibenclamide) and Glimepiride.
Biological Activity
Glipizide-d11 is a stable isotope-labeled derivative of glipizide, a widely used sulfonylurea for managing type 2 diabetes mellitus. This article explores the biological activity of this compound, including its pharmacodynamics, pharmacokinetics, and clinical implications based on diverse research findings.
Overview of Glipizide
Glipizide functions primarily as an insulin secretagogue, stimulating insulin release from pancreatic beta cells. It achieves this by binding to the sulfonylurea receptor on these cells, leading to the closure of ATP-sensitive potassium channels and subsequent depolarization. This process opens voltage-sensitive calcium channels, allowing calcium influx that triggers insulin exocytosis .
The biological activity of this compound mirrors that of its parent compound, glipizide. Key mechanisms include:
- Insulin Secretion : Stimulates insulin release from pancreatic beta cells.
- Extrapancreatic Effects : Enhances glucose uptake in skeletal muscles and promotes hepatic insulin sensitivity.
- Inhibition of Lipolysis : Reduces lipid breakdown in both liver and adipose tissues, contributing to overall glucose homeostasis .
Pharmacokinetics
The pharmacokinetic profile of this compound is critical for understanding its biological activity:
- Absorption : Glipizide is rapidly absorbed with nearly complete bioavailability. Peak plasma concentrations occur within 6 to 12 hours post-administration .
- Distribution : The volume of distribution is approximately 10 L, indicating significant tissue uptake.
- Protein Binding : Glipizide exhibits high protein binding (approximately 90%), which may influence its therapeutic efficacy and duration of action .
Efficacy and Safety
A study assessing the efficacy of glipizide extended-release formulations demonstrated significant reductions in fasting plasma glucose (FPG) and glycosylated hemoglobin (HbA1c) levels across various doses. For instance:
Dose (mg) | Reduction in FPG (mg/dL) | Reduction in HbA1c (%) |
---|---|---|
5 | -57 | -1.50 |
20 | -74 | -1.82 |
These findings indicate that glipizide effectively lowers blood glucose levels without adverse effects on lipid profiles or body weight .
Case Study: Drug-Induced Acute Pancreatitis
A notable case report highlighted a patient who developed acute pancreatitis while on a regimen that included glipizide. The patient experienced severe abdominal pain and elevated serum lipase levels but improved after discontinuing the medication. This case underscores the importance of monitoring potential adverse effects associated with glipizide therapy, especially when combined with other agents like dulaglutide .
Comparative Analysis with Other Antidiabetic Agents
This compound's mechanism and effects can be compared with other antidiabetic medications:
Drug Class | Mechanism | Common Side Effects |
---|---|---|
Sulfonylureas | Insulin secretion stimulation | Hypoglycemia, weight gain |
DPP-4 Inhibitors | Incretin enhancement | Gastrointestinal symptoms |
GLP-1 Agonists | Appetite suppression, insulin secretion | Nausea, pancreatitis risk |
While sulfonylureas like glipizide are effective for lowering blood sugar, they carry risks such as hypoglycemia and potential weight gain .
Q & A
Basic Research Questions
Q. What are the key structural and functional distinctions between Glipizide and Glipizide-d11, and how do these differences influence experimental design?
this compound, a deuterated analog of Glipizide, replaces 11 hydrogen atoms with deuterium to enhance metabolic stability while retaining pharmacological activity. The isotopic substitution reduces the rate of CYP450-mediated metabolism, enabling precise pharmacokinetic tracking in vivo. Methodologically, researchers must validate deuterium incorporation using high-resolution mass spectrometry (HRMS) or nuclear magnetic resonance (NMR) spectroscopy . Experimental designs should include control groups using non-deuterated Glipizide to isolate isotope effects on bioavailability and efficacy.
Q. How should researchers validate the purity and stability of this compound in preclinical studies?
Purity validation requires chromatographic techniques (e.g., HPLC or UPLC) coupled with UV/Vis or tandem mass spectrometry (MS/MS) to detect impurities ≤0.1%. Stability studies under varied conditions (pH, temperature, light) should follow ICH guidelines Q1A(R2). For deuterated compounds, isotopic purity must be confirmed via isotopic abundance ratios (e.g., d11 ≥ 98%) using HRMS .
Q. What in vitro models are optimal for assessing this compound’s mechanism of action on pancreatic β-cells?
Use pancreatic β-cell lines (e.g., INS-1 or MIN6) to evaluate KATP channel inhibition via patch-clamp electrophysiology. Measure insulin secretion under glucose-stimulated conditions and compare dose-response curves between Glipizide and this compound to identify isotope-specific effects. Include ATP-sensitive potassium channel blockers (e.g., tolbutamide) as positive controls .
Advanced Research Questions
Q. How can isotope effects in this compound complicate pharmacokinetic (PK) data interpretation, and what statistical methods mitigate these challenges?
Deuterium’s kinetic isotope effect (KIE) may prolong half-life (t½) and alter clearance rates. To disentangle isotope-specific outcomes, employ compartmental PK modeling with Bayesian hierarchical frameworks to account for inter-subject variability. Pair this with metabolite profiling (LC-MS/MS) to track deuterium retention in major metabolic pathways (e.g., hydroxylation, glucuronidation) .
Q. What experimental strategies resolve contradictions in efficacy data between this compound and non-deuterated analogs in diabetic rodent models?
Contradictions often arise from differences in metabolic enzyme expression (e.g., CYP2C9 polymorphisms). Address this by:
- Conducting enzyme kinetics assays with recombinant CYP isoforms.
- Using genetic knockout models (e.g., CYP2C9-null mice) to isolate metabolic pathways.
- Applying mixed-effects regression models to adjust for covariates like age, sex, and genetic background .
Q. How should researchers design isotope tracer studies to quantify this compound’s tissue-specific biodistribution?
Use autoradiography or PET/CT imaging with radiolabeled this compound (e.g., ¹⁴C or ³H). For non-radioactive approaches, employ spatially resolved mass spectrometry imaging (MSI) to map deuterium distribution in tissues. Normalize data to total protein content or internal standards (e.g., deuterated internal controls) to correct for matrix effects .
Q. Methodological Considerations
Q. What documentation standards ensure reproducibility in this compound studies?
Adhere to FAIR (Findable, Accessible, Interoperable, Reusable) principles:
- Publish raw NMR/MS spectra, chromatograms, and kinetic datasets in supplementary materials.
- Detail isotopic synthesis protocols (e.g., reaction conditions, catalysts, purification steps).
- Archive computational workflows (e.g., PK/PD modeling scripts) on platforms like GitHub or Zenodo .
Q. How can conflicting data on this compound’s hypoglycemic risk be analyzed in meta-analyses?
Perform systematic reviews using PRISMA guidelines. Apply random-effects meta-regression to assess heterogeneity sources (e.g., dosing regimens, animal strain differences). Use sensitivity analyses to exclude outliers and GRADE criteria to evaluate evidence quality .
Q. Data Presentation Guidelines
Parameter | Analytical Technique | Acceptance Criteria |
---|---|---|
Isotopic Purity | HRMS | d11 abundance ≥98% |
Metabolic Stability | LC-MS/MS | t½ ≥2x non-deuterated control |
KATP Channel IC50 | Patch-clamp | ≤10 nM (consistent with parent) |
Properties
IUPAC Name |
5-methyl-N-[2-[4-[(1,2,2,3,3,4,4,5,5,6,6-undecadeuteriocyclohexyl)carbamoylsulfamoyl]phenyl]ethyl]pyrazine-2-carboxamide | |
---|---|---|
Source | PubChem | |
URL | https://pubchem.ncbi.nlm.nih.gov | |
Description | Data deposited in or computed by PubChem | |
InChI |
InChI=1S/C21H27N5O4S/c1-15-13-24-19(14-23-15)20(27)22-12-11-16-7-9-18(10-8-16)31(29,30)26-21(28)25-17-5-3-2-4-6-17/h7-10,13-14,17H,2-6,11-12H2,1H3,(H,22,27)(H2,25,26,28)/i2D2,3D2,4D2,5D2,6D2,17D | |
Source | PubChem | |
URL | https://pubchem.ncbi.nlm.nih.gov | |
Description | Data deposited in or computed by PubChem | |
InChI Key |
ZJJXGWJIGJFDTL-KVEFGMAKSA-N | |
Source | PubChem | |
URL | https://pubchem.ncbi.nlm.nih.gov | |
Description | Data deposited in or computed by PubChem | |
Canonical SMILES |
CC1=CN=C(C=N1)C(=O)NCCC2=CC=C(C=C2)S(=O)(=O)NC(=O)NC3CCCCC3 | |
Source | PubChem | |
URL | https://pubchem.ncbi.nlm.nih.gov | |
Description | Data deposited in or computed by PubChem | |
Isomeric SMILES |
[2H]C1(C(C(C(C(C1([2H])[2H])([2H])[2H])([2H])NC(=O)NS(=O)(=O)C2=CC=C(C=C2)CCNC(=O)C3=NC=C(N=C3)C)([2H])[2H])([2H])[2H])[2H] | |
Source | PubChem | |
URL | https://pubchem.ncbi.nlm.nih.gov | |
Description | Data deposited in or computed by PubChem | |
Molecular Formula |
C21H27N5O4S | |
Source | PubChem | |
URL | https://pubchem.ncbi.nlm.nih.gov | |
Description | Data deposited in or computed by PubChem | |
Molecular Weight |
456.6 g/mol | |
Source | PubChem | |
URL | https://pubchem.ncbi.nlm.nih.gov | |
Description | Data deposited in or computed by PubChem | |
Disclaimer and Information on In-Vitro Research Products
Please be aware that all articles and product information presented on BenchChem are intended solely for informational purposes. The products available for purchase on BenchChem are specifically designed for in-vitro studies, which are conducted outside of living organisms. In-vitro studies, derived from the Latin term "in glass," involve experiments performed in controlled laboratory settings using cells or tissues. It is important to note that these products are not categorized as medicines or drugs, and they have not received approval from the FDA for the prevention, treatment, or cure of any medical condition, ailment, or disease. We must emphasize that any form of bodily introduction of these products into humans or animals is strictly prohibited by law. It is essential to adhere to these guidelines to ensure compliance with legal and ethical standards in research and experimentation.