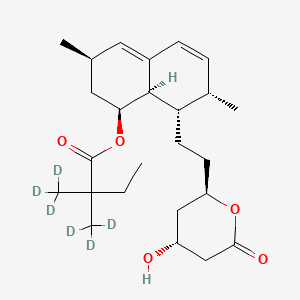
Simvastatin-d6
- Click on QUICK INQUIRY to receive a quote from our team of experts.
- With the quality product at a COMPETITIVE price, you can focus more on your research.
Overview
Description
Simvastatin-d6 is a deuterated form of simvastatin, a lipid-lowering medication belonging to the statin class. It is primarily used as an internal standard for the quantification of simvastatin in various analytical applications. The compound is characterized by the presence of six deuterium atoms, which replace six hydrogen atoms in the simvastatin molecule, providing enhanced stability and traceability in mass spectrometry analyses .
Mechanism of Action
Target of Action
Simvastatin-d6, like other statins, primarily targets the enzyme hydroxymethylglutaryl-coenzyme A (HMG-CoA) Reductase . This enzyme plays a crucial role in the mevalonate pathway , which is responsible for the endogenous production of cholesterol in the liver . By inhibiting HMG-CoA reductase, this compound effectively reduces the synthesis of cholesterol, thereby lowering lipid levels and reducing the risk of cardiovascular events .
Mode of Action
This compound is a prodrug that is hydrolyzed in vivo to generate an active metabolite structurally similar to HMG-CoA . This metabolite competitively inhibits HMG-CoA reductase, preventing the conversion of HMG-CoA to mevalonic acid . This inhibition disrupts the mevalonate pathway, leading to a decrease in the production of cholesterol and other compounds involved in lipid metabolism and transport, including low-density lipoprotein (LDL) and very low-density lipoprotein (VLDL) .
Biochemical Pathways
The primary biochemical pathway affected by this compound is the mevalonate pathway . By inhibiting HMG-CoA reductase, this compound disrupts the conversion of HMG-CoA to mevalonic acid, a key step in this pathway . This leads to a decrease in the production of several compounds involved in lipid metabolism and transport, including cholesterol, LDL, and VLDL . Additionally, this compound can also influence the expression of genes related to de novo synthesis of triacylglycerols and phospholipids .
Pharmacokinetics
This compound, like other statins, exhibits specific ADME (Absorption, Distribution, Metabolism, and Excretion) properties . It is absorbed and distributed in the body, where it is metabolized primarily by cytochrome P450 (CYP) 3A4 . The bioavailability of simvastatin is relatively low, around 5% . It is worth noting that the lipophilicity of simvastatin allows it to enter cells directly .
Result of Action
The primary molecular effect of this compound is the reduction of cholesterol levels in the body . This results in a decrease in the levels of LDL and VLDL, often referred to as “bad cholesterol” due to their association with cardiovascular disease . On a cellular level, this compound has been shown to induce pyroptosis, a form of programmed cell death, in certain cancer cells . It also leads to the accumulation of cytosolic lipid droplets within cells, which might contribute to its antiproliferative effects .
Action Environment
Environmental factors can influence the action of this compound. For instance, the presence of certain bile acids can augment the bioaccumulation of this compound in probiotic bacteria . Moreover, this compound has been detected in various waters, indicating its potential as an environmental pollutant . This environmental presence could potentially affect non-target aquatic organisms, disrupting their antioxidant-related genes
Biochemical Analysis
Biochemical Properties
Simvastatin-d6 interacts with various enzymes and proteins in the body. It is a competitive inhibitor of HMG-CoA reductase, an enzyme that catalyzes the conversion of HMG-CoA to mevalonic acid, a key step in cholesterol synthesis . By inhibiting this enzyme, this compound reduces the production of cholesterol in the body .
Cellular Effects
This compound has significant effects on various types of cells and cellular processes. It has been shown to increase the intracellular content of lipid droplets in both non-malignant and cancer cells . It also improves endothelial cell function by inhibiting endothelial-to-mesenchymal transition (EndMT) through an epigenetic regulatory mechanism .
Molecular Mechanism
This compound exerts its effects at the molecular level through various mechanisms. It is a prodrug that is hydrolyzed in vivo to generate the active simvastatin acid, which is structurally similar to HMG-CoA . This allows it to competitively inhibit HMG-CoA reductase, thereby reducing cholesterol synthesis . Additionally, this compound has been shown to suppress TLR4/MyD88/NF-ĸB signaling, causing an immune response shift to an anti-inflammatory response .
Temporal Effects in Laboratory Settings
Over time, this compound has been observed to have varying effects in laboratory settings. For instance, long-term exposure to statins, including this compound, has been associated with a statistically significant increase in the risk of new onset type 2 diabetes .
Dosage Effects in Animal Models
The effects of this compound vary with different dosages in animal models. For instance, in a study on the effects of simvastatin on neurodevelopmental disorders, it was found that simvastatin at a dose of 40 mg should be avoided in decompensated cirrhosis, while simvastatin 20 mg or other statins based on small study sample size were found to be safe .
Metabolic Pathways
This compound is involved in the mevalonate pathway, which is responsible for the production of several compounds involved in lipid metabolism and transport, including cholesterol, low-density lipoprotein (LDL), and very low-density lipoprotein (VLDL) .
Transport and Distribution
This compound is transported and distributed within cells and tissues. It is known to be transported into bacteria cells leading to a drug bioaccumulation over time, which is augmented upon addition of bile acids .
Subcellular Localization
Given its role in inhibiting HMG-CoA reductase, an enzyme located in the endoplasmic reticulum, it can be inferred that this compound may localize to this organelle to exert its effects .
Preparation Methods
Synthetic Routes and Reaction Conditions
The synthesis of simvastatin-d6 involves the incorporation of deuterium atoms into the simvastatin molecule. This can be achieved through various methods, including deuterium exchange reactions and the use of deuterated reagents. One common approach is the hydrogen-deuterium exchange reaction, where simvastatin is treated with deuterium gas in the presence of a catalyst, resulting in the replacement of hydrogen atoms with deuterium atoms .
Industrial Production Methods
Industrial production of this compound typically involves large-scale deuterium exchange reactionsThe reaction conditions, such as temperature, pressure, and catalyst type, are optimized to achieve high yields and purity of the deuterated product .
Chemical Reactions Analysis
Types of Reactions
Simvastatin-d6 undergoes various chemical reactions, including oxidation, reduction, and substitution reactions. These reactions are essential for the compound’s analytical applications and its role as an internal standard.
Common Reagents and Conditions
Oxidation: this compound can be oxidized using oxidizing agents such as potassium permanganate or hydrogen peroxide under controlled conditions.
Reduction: Reduction reactions can be carried out using reducing agents like sodium borohydride or lithium aluminum hydride.
Major Products
The major products formed from these reactions depend on the specific reagents and conditions used. For example, oxidation of this compound can yield oxidized derivatives, while reduction can produce reduced forms of the compound. Substitution reactions result in the formation of substituted derivatives with different functional groups .
Scientific Research Applications
Simvastatin-d6 has a wide range of scientific research applications, particularly in the fields of chemistry, biology, medicine, and industry. Some of its key applications include:
Analytical Chemistry: Used as an internal standard in mass spectrometry for the quantification of simvastatin and its metabolites.
Pharmacokinetics: Employed in pharmacokinetic studies to track the absorption, distribution, metabolism, and excretion of simvastatin in biological systems.
Biomedical Research: Investigated for its potential therapeutic effects in various diseases, including cardiovascular diseases, cancer, and neurodegenerative disorders.
Industrial Applications: Utilized in the development and quality control of pharmaceutical formulations containing simvastatin .
Comparison with Similar Compounds
Simvastatin-d6 is unique due to its deuterated nature, which provides enhanced stability and traceability in analytical applications. Similar compounds include other deuterated statins and non-deuterated statins such as:
Atorvastatin: Another statin used to lower cholesterol levels, but without deuterium atoms.
Pravastatin: A statin with a different chemical structure and pharmacokinetic profile.
Rosuvastatin: Known for its high potency in reducing low-density lipoprotein cholesterol levels.
Lovastatin: A naturally occurring statin with similar lipid-lowering effects
This compound stands out due to its specific use as an internal standard in analytical chemistry, providing accurate and reliable quantification of simvastatin in various research and industrial applications.
Properties
IUPAC Name |
[(1S,3R,7S,8S,8aR)-8-[2-[(2R,4R)-4-hydroxy-6-oxooxan-2-yl]ethyl]-3,7-dimethyl-1,2,3,7,8,8a-hexahydronaphthalen-1-yl] 2,2-bis(trideuteriomethyl)butanoate |
Source
|
---|---|---|
Source | PubChem | |
URL | https://pubchem.ncbi.nlm.nih.gov | |
Description | Data deposited in or computed by PubChem | |
InChI |
InChI=1S/C25H38O5/c1-6-25(4,5)24(28)30-21-12-15(2)11-17-8-7-16(3)20(23(17)21)10-9-19-13-18(26)14-22(27)29-19/h7-8,11,15-16,18-21,23,26H,6,9-10,12-14H2,1-5H3/t15-,16-,18+,19+,20-,21-,23-/m0/s1/i4D3,5D3 |
Source
|
Source | PubChem | |
URL | https://pubchem.ncbi.nlm.nih.gov | |
Description | Data deposited in or computed by PubChem | |
InChI Key |
RYMZZMVNJRMUDD-QDGXURMLSA-N |
Source
|
Source | PubChem | |
URL | https://pubchem.ncbi.nlm.nih.gov | |
Description | Data deposited in or computed by PubChem | |
Canonical SMILES |
CCC(C)(C)C(=O)OC1CC(C=C2C1C(C(C=C2)C)CCC3CC(CC(=O)O3)O)C |
Source
|
Source | PubChem | |
URL | https://pubchem.ncbi.nlm.nih.gov | |
Description | Data deposited in or computed by PubChem | |
Isomeric SMILES |
[2H]C([2H])([2H])C(CC)(C(=O)O[C@H]1C[C@H](C=C2[C@H]1[C@H]([C@H](C=C2)C)CC[C@@H]3C[C@H](CC(=O)O3)O)C)C([2H])([2H])[2H] |
Source
|
Source | PubChem | |
URL | https://pubchem.ncbi.nlm.nih.gov | |
Description | Data deposited in or computed by PubChem | |
Molecular Formula |
C25H38O5 |
Source
|
Source | PubChem | |
URL | https://pubchem.ncbi.nlm.nih.gov | |
Description | Data deposited in or computed by PubChem | |
Molecular Weight |
424.6 g/mol |
Source
|
Source | PubChem | |
URL | https://pubchem.ncbi.nlm.nih.gov | |
Description | Data deposited in or computed by PubChem | |
Q1: Why is Simvastatin-d6 used in the LC-MS-MS method described in the research paper []?
A1: this compound serves as an internal standard in the liquid chromatography-tandem mass spectrometry (LC-MS-MS) method developed by the researchers []. Internal standards are crucial for accurate quantification in analytical chemistry, particularly when analyzing complex biological samples like plasma.
Disclaimer and Information on In-Vitro Research Products
Please be aware that all articles and product information presented on BenchChem are intended solely for informational purposes. The products available for purchase on BenchChem are specifically designed for in-vitro studies, which are conducted outside of living organisms. In-vitro studies, derived from the Latin term "in glass," involve experiments performed in controlled laboratory settings using cells or tissues. It is important to note that these products are not categorized as medicines or drugs, and they have not received approval from the FDA for the prevention, treatment, or cure of any medical condition, ailment, or disease. We must emphasize that any form of bodily introduction of these products into humans or animals is strictly prohibited by law. It is essential to adhere to these guidelines to ensure compliance with legal and ethical standards in research and experimentation.