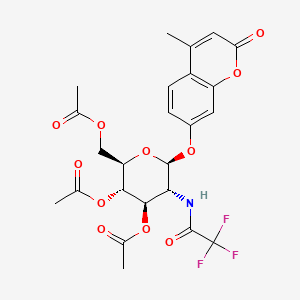
4-Methylumbelliferyl 3,4,6-tri-O-acetyl-2-deoxy-2-trifluoroacetamido-b-D-glucopyranoside
Overview
Description
4-Methylumbelliferyl 3,4,6-tri-O-acetyl-2-deoxy-2-trifluoroacetamido-b-D-glucopyranoside is a complex glycoside compound known for its utility in biochemical assays and research applications. This compound is characterized by its intricate molecular structure, which includes a 4-methylumbelliferyl group and a modified glucopyranoside moiety.
Mechanism of Action
Target of Action
The primary targets of 4-Methylumbelliferyl 3,4,6-tri-O-acetyl-2-deoxy-2-trifluoroacetamido-b-D-glucopyranoside are enzymes involved in glycosylation processes . These enzymes play a crucial role in the synthesis and breakdown of glycoproteins and glycolipids, which are essential components of cellular membranes and play a significant role in cell-cell interactions.
Mode of Action
This compound acts as a substrate for these enzymes . It is designed to mimic the natural substrates of the enzymes, allowing it to bind to the enzyme’s active site. Once bound, the enzyme catalyzes a reaction that results in the cleavage of the 4-Methylumbelliferyl moiety from the rest of the molecule. This cleavage results in the release of a fluorescent signal, which can be detected and quantified .
Biochemical Pathways
The biochemical pathways affected by this compound are primarily those involved in the metabolism of carbohydrates . Specifically, it is used in the study of chitinase activity, an enzyme involved in the breakdown of chitin, a long-chain polymer of N-acetylglucosamine, a derivative of glucose .
Result of Action
The primary result of the action of this compound is the generation of a fluorescent signal upon cleavage by the target enzyme . This allows for the detection and quantification of enzyme activity, providing valuable information about the enzyme’s function and potential role in disease processes.
Preparation Methods
Synthetic Routes and Reaction Conditions: The synthesis of 4-Methylumbelliferyl 3,4,6-tri-O-acetyl-2-deoxy-2-trifluoroacetamido-b-D-glucopyranoside typically involves multiple steps, starting with the protection of the hydroxyl groups on the glucopyranoside ring. This is followed by the introduction of the trifluoroacetamido group and the 4-methylumbelliferyl moiety. The reaction conditions often require the use of strong bases and protecting groups to ensure the selective formation of the desired product.
Industrial Production Methods: In an industrial setting, the production of this compound is scaled up using reactors that allow for precise control of temperature, pressure, and reagent concentrations. The process involves the use of automated systems to ensure consistency and purity of the final product. Quality control measures, such as high-performance liquid chromatography (HPLC), are employed to verify the identity and purity of the compound.
Chemical Reactions Analysis
Types of Reactions: This compound undergoes various types of chemical reactions, including oxidation, reduction, and substitution reactions. The presence of multiple functional groups allows for a wide range of chemical transformations.
Common Reagents and Conditions:
Oxidation: Common oxidizing agents include hydrogen peroxide and chromium(VI) compounds.
Reduction: Reducing agents such as lithium aluminum hydride (LiAlH4) are often used.
Substitution: Nucleophilic substitution reactions are facilitated by strong bases like sodium hydride (NaH).
Major Products Formed: The major products formed from these reactions include oxidized derivatives, reduced forms, and various substituted analogs, depending on the specific reagents and conditions used.
Scientific Research Applications
Chemistry: In chemistry, this compound is used as a substrate in enzyme assays to study glycosidase activities. It serves as a fluorogenic substrate, emitting fluorescence upon cleavage by glycosidases, which makes it valuable for detecting enzyme activity.
Biology: In biological research, it is employed to investigate carbohydrate metabolism and glycosidase enzyme functions. Its use in enzyme assays helps in understanding the mechanisms of glycosidase inhibitors and their potential therapeutic applications.
Medicine: The compound is utilized in the development of diagnostic tools and therapeutic agents. Its ability to act as a substrate for glycosidases makes it useful in identifying diseases related to carbohydrate metabolism disorders.
Industry: In the industrial sector, it is used in the production of biochemical reagents and research kits. Its role in enzyme assays ensures the quality and efficacy of these products.
Comparison with Similar Compounds
4-Methylumbelliferyl 2-acetamido-3,4,6-tri-O-acetyl-2-deoxy-b-D-galactopyranoside: Used in biomedical research for studying chitinase activity.
4-Methylumbelliferyl 2-acetamido-3-O-(2,3,4-tri-O-acetyl-a-L-fucopyranosyl)-4-O-(2,3,4,6-tetra-O-acetyl-\beta-D-galactopyranosyl)-6-O-acetyl-2-deoxy-\beta-D-glucopyranoside: Employed as a substrate for enzyme assays in glycosidase activity detection.
Uniqueness: The uniqueness of 4-Methylumbelliferyl 3,4,6-tri-O-acetyl-2-deoxy-2-trifluoroacetamido-b-D-glucopyranoside lies in its specific structural features, which make it particularly suitable for detecting glycosidase activities. Its trifluoroacetamido group and 4-methylumbelliferyl moiety provide distinct advantages in terms of sensitivity and specificity in enzyme assays.
Properties
IUPAC Name |
[(2R,3S,4R,5R,6S)-3,4-diacetyloxy-6-(4-methyl-2-oxochromen-7-yl)oxy-5-[(2,2,2-trifluoroacetyl)amino]oxan-2-yl]methyl acetate | |
---|---|---|
Source | PubChem | |
URL | https://pubchem.ncbi.nlm.nih.gov | |
Description | Data deposited in or computed by PubChem | |
InChI |
InChI=1S/C24H24F3NO11/c1-10-7-18(32)38-16-8-14(5-6-15(10)16)37-22-19(28-23(33)24(25,26)27)21(36-13(4)31)20(35-12(3)30)17(39-22)9-34-11(2)29/h5-8,17,19-22H,9H2,1-4H3,(H,28,33)/t17-,19-,20-,21-,22-/m1/s1 | |
Source | PubChem | |
URL | https://pubchem.ncbi.nlm.nih.gov | |
Description | Data deposited in or computed by PubChem | |
InChI Key |
MGLRSUPYLXVODY-MSEOUXRUSA-N | |
Source | PubChem | |
URL | https://pubchem.ncbi.nlm.nih.gov | |
Description | Data deposited in or computed by PubChem | |
Canonical SMILES |
CC1=CC(=O)OC2=C1C=CC(=C2)OC3C(C(C(C(O3)COC(=O)C)OC(=O)C)OC(=O)C)NC(=O)C(F)(F)F | |
Source | PubChem | |
URL | https://pubchem.ncbi.nlm.nih.gov | |
Description | Data deposited in or computed by PubChem | |
Isomeric SMILES |
CC1=CC(=O)OC2=C1C=CC(=C2)O[C@H]3[C@@H]([C@H]([C@@H]([C@H](O3)COC(=O)C)OC(=O)C)OC(=O)C)NC(=O)C(F)(F)F | |
Source | PubChem | |
URL | https://pubchem.ncbi.nlm.nih.gov | |
Description | Data deposited in or computed by PubChem | |
Molecular Formula |
C24H24F3NO11 | |
Source | PubChem | |
URL | https://pubchem.ncbi.nlm.nih.gov | |
Description | Data deposited in or computed by PubChem | |
DSSTOX Substance ID |
DTXSID301106953 | |
Record name | 2H-1-Benzopyran-2-one, 4-methyl-7-[[3,4,6-tri-O-acetyl-2-deoxy-2-[(trifluoroacetyl)amino]-β-D-glucopyranosyl]oxy]- | |
Source | EPA DSSTox | |
URL | https://comptox.epa.gov/dashboard/DTXSID301106953 | |
Description | DSSTox provides a high quality public chemistry resource for supporting improved predictive toxicology. | |
Molecular Weight |
559.4 g/mol | |
Source | PubChem | |
URL | https://pubchem.ncbi.nlm.nih.gov | |
Description | Data deposited in or computed by PubChem | |
CAS No. |
137686-93-2 | |
Record name | 2H-1-Benzopyran-2-one, 4-methyl-7-[[3,4,6-tri-O-acetyl-2-deoxy-2-[(trifluoroacetyl)amino]-β-D-glucopyranosyl]oxy]- | |
Source | CAS Common Chemistry | |
URL | https://commonchemistry.cas.org/detail?cas_rn=137686-93-2 | |
Description | CAS Common Chemistry is an open community resource for accessing chemical information. Nearly 500,000 chemical substances from CAS REGISTRY cover areas of community interest, including common and frequently regulated chemicals, and those relevant to high school and undergraduate chemistry classes. This chemical information, curated by our expert scientists, is provided in alignment with our mission as a division of the American Chemical Society. | |
Explanation | The data from CAS Common Chemistry is provided under a CC-BY-NC 4.0 license, unless otherwise stated. | |
Record name | 2H-1-Benzopyran-2-one, 4-methyl-7-[[3,4,6-tri-O-acetyl-2-deoxy-2-[(trifluoroacetyl)amino]-β-D-glucopyranosyl]oxy]- | |
Source | EPA DSSTox | |
URL | https://comptox.epa.gov/dashboard/DTXSID301106953 | |
Description | DSSTox provides a high quality public chemistry resource for supporting improved predictive toxicology. | |
Disclaimer and Information on In-Vitro Research Products
Please be aware that all articles and product information presented on BenchChem are intended solely for informational purposes. The products available for purchase on BenchChem are specifically designed for in-vitro studies, which are conducted outside of living organisms. In-vitro studies, derived from the Latin term "in glass," involve experiments performed in controlled laboratory settings using cells or tissues. It is important to note that these products are not categorized as medicines or drugs, and they have not received approval from the FDA for the prevention, treatment, or cure of any medical condition, ailment, or disease. We must emphasize that any form of bodily introduction of these products into humans or animals is strictly prohibited by law. It is essential to adhere to these guidelines to ensure compliance with legal and ethical standards in research and experimentation.