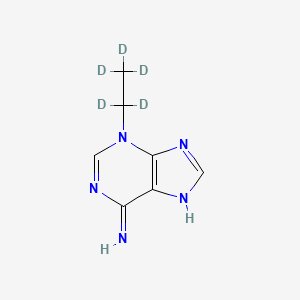
3-Ethyl-d5-adenine
Overview
Description
3-Ethyl-d5-adenine (CAS: 147028-85-1) is a deuterated derivative of adenine, a purine nucleobase critical to genetic and metabolic processes. Its molecular formula is C₇H₄D₅N₅, with a molecular weight of 168.21 g/mol . The compound features an ethyl group substituted at the 3-position of the adenine ring, with five deuterium atoms replacing hydrogen in the ethyl moiety (denoted as -d5). This isotopic labeling makes it valuable in pharmacokinetic and metabolic studies, where deuterium incorporation enhances stability and traceability in mass spectrometry .
Preparation Methods
Synthetic Routes and Reaction Conditions
The synthesis of 3-Ethyl-d5-adenine typically involves the alkylation of adenine with deuterated ethyl groups. The reaction conditions often require a deuterated ethylating agent and a suitable base to facilitate the substitution reaction. The process may involve multiple steps, including purification and isolation of the final product to ensure high purity and isotopic enrichment.
Industrial Production Methods
Industrial production of this compound follows similar synthetic routes but on a larger scale. The process involves stringent quality control measures to ensure the isotopic purity and consistency of the product. The use of automated systems and advanced analytical techniques helps in maintaining the quality and efficiency of the production process.
Chemical Reactions Analysis
Types of Reactions
3-Ethyl-d5-adenine undergoes various chemical reactions, including:
Oxidation: The compound can be oxidized under specific conditions to form corresponding oxidized products.
Reduction: Reduction reactions can be performed to modify the functional groups attached to the adenine ring.
Substitution: The ethyl group can be substituted with other functional groups using appropriate reagents.
Common Reagents and Conditions
Oxidation: Common oxidizing agents include hydrogen peroxide and potassium permanganate.
Reduction: Reducing agents such as sodium borohydride and lithium aluminum hydride are used.
Substitution: Various alkylating agents and bases are employed for substitution reactions.
Major Products Formed
The major products formed from these reactions depend on the specific reagents and conditions used. For example, oxidation may yield hydroxylated derivatives, while substitution reactions can produce a wide range of functionalized adenine derivatives.
Scientific Research Applications
Chemistry
3-Ethyl-d5-adenine serves as a model compound to study the effects of alkylation on nucleobases. Researchers investigate how the ethyl group influences chemical reactivity and interactions with other molecules. This understanding contributes to broader insights into nucleobase behavior under various chemical conditions.
Biology
In biological research, this compound is crucial for studying DNA repair mechanisms. Alkylated bases can lead to mutations, prompting cellular repair processes. The compound's role in mutagenesis is significant for understanding genetic variability and the underlying mechanisms that maintain genomic integrity.
Medicine
The potential implications of this compound in cancer research are notable. Alkylating agents are known to induce mutations that can contribute to cancer development. Studies have shown that compounds like this compound can lead to G/C to A/T transitions during DNA replication, which may activate oncogenic pathways.
Case Studies and Findings
-
Cigarette Smoke Exposure:
Elevated levels of this compound have been detected in the urine of smokers, indicating that cigarette smoke contains DNA-alkylating agents. This exposure correlates with increased levels of N-terminal N-ethylvaline in hemoglobin, suggesting systemic effects from alkylation. -
Ethyl Methanesulfonate Mutagenesis:
Ethyl methanesulfonate (EMS), a known mutagen, has been shown to induce mutations through the formation of this compound. Studies involving Arabidopsis and rice revealed a variety of mutations predominantly characterized by G/C to A/T transitions due to mispairing facilitated by this compound.
Activity Type | Description |
---|---|
Mutagenesis | Induces G/C to A/T transitions during DNA replication |
DNA Repair | Activates cellular repair pathways due to alkylation-induced damage |
Implications in Cancer Research
The mutagenic potential of this compound raises concerns regarding its role in cancer development. The ability to induce DNA damage positions this compound as a significant focus in cancer biology research, where it contributes to understanding genomic instability—a hallmark characteristic of cancer cells.
Mechanism of Action
The mechanism of action of 3-Ethyl-d5-adenine involves its incorporation into nucleic acids, where it can be used to study various biochemical processes. The deuterium labeling allows for precise tracking and analysis of the compound in biological systems. The molecular targets and pathways involved include DNA and RNA synthesis, as well as enzymatic reactions related to nucleic acid metabolism.
Comparison with Similar Compounds
Key Properties:
- Solubility: Slightly soluble in methanol .
- Stability: No explicit stability data are available, but deuterated analogs typically exhibit slower metabolic degradation due to the kinetic isotope effect .
- Applications : Used as a stable isotope-labeled internal standard in organic synthesis, drug metabolism studies, and biochemical assays .
Adenine derivatives are widely utilized in research and pharmacology. Below is a detailed comparison of 3-Ethyl-d5-adenine with structurally related compounds:
Table 1: Structural and Functional Comparison
Substituent Position and Functional Impact
- This compound: The ethyl-d5 group at the 3-position introduces steric bulk and isotopic labeling, making it less reactive than non-deuterated analogs. This substitution minimizes metabolic cleavage, enhancing its utility in tracer studies .
- 9-(2-Hydroxyethyl)adenine: The 9-position hydroxyethyl group increases hydrophilicity, improving water solubility. This compound is often used to mimic adenosine in enzyme-binding assays .
- Adenine Hemisulfate Dihydrate : A salt form of adenine, it enhances stability in aqueous solutions, making it ideal for cell culture and nucleotide synthesis .
Isotopic Labeling and Stability
- This compound vs. 3-Methyl-d3-adenine : The ethyl-d5 group provides greater isotopic mass shift (5 deuteriums vs. 3), improving detection sensitivity in mass spectrometry. However, the longer ethyl chain may reduce solubility compared to the methyl analog .
Solubility and Handling
- This compound: Limited methanol solubility necessitates careful formulation in experimental protocols .
- 9-(2-Hydroxyethyl)adenine : Higher water solubility due to the polar hydroxyethyl group simplifies use in biological buffers .
Biological Activity
3-Ethyl-d5-adenine is a modified adenine nucleoside that has garnered interest in biochemical research due to its potential biological activity, particularly in the context of cellular metabolism and signaling pathways. This article reviews the biological properties of this compound, focusing on its mechanisms of action, effects on cellular processes, and implications for therapeutic applications.
Chemical Structure and Properties
This compound is a derivative of adenine, characterized by an ethyl group substitution at the 3-position of the purine ring. The incorporation of deuterium at specific positions enhances its stability and allows for precise tracking in metabolic studies. The molecular formula is C₇H₉D₅N₄O₃, and it is often used as a tracer in metabolic studies due to its unique isotopic labeling.
1. Interaction with Adenosine Receptors:
this compound has been shown to interact with adenosine receptors, which play crucial roles in various physiological processes including neurotransmission, inflammation, and cardiovascular function. Studies indicate that this compound can modulate receptor activity, potentially influencing downstream signaling pathways such as cyclic AMP (cAMP) production.
2. Inhibition of Adenylyl Cyclase:
Research has demonstrated that this compound can inhibit adenylyl cyclase activity, which is responsible for converting ATP to cAMP. This inhibition can affect cellular responses to hormones and neurotransmitters, thereby altering metabolic pathways and gene expression .
Biological Activities
1. Effects on Cellular Metabolism:
In vitro studies have shown that this compound influences metabolic rates in various cell lines. For instance, it has been observed to enhance glycolytic activity while simultaneously decreasing oxidative phosphorylation efficiency. This dual effect suggests a shift towards anaerobic metabolism under certain conditions, which may be beneficial in hypoxic environments .
2. Role in Cancer Research:
The compound's ability to modulate cellular energy metabolism has implications for cancer research. By altering ATP levels and cAMP signaling, this compound may affect tumor growth and survival mechanisms. Preliminary studies indicate that it could serve as a potential therapeutic agent by sensitizing cancer cells to chemotherapy through metabolic reprogramming .
Case Studies
Case Study 1: Metabolic Profiling in Cancer Cells
A recent study investigated the effects of this compound on breast cancer cell lines. The results showed that treatment with the compound led to a significant reduction in cell viability and increased apoptosis markers compared to control groups. This suggests that this compound may enhance the efficacy of existing chemotherapeutic agents by targeting metabolic vulnerabilities in cancer cells.
Case Study 2: Neuroprotective Effects
Another study explored the neuroprotective effects of this compound in models of neurodegeneration. The findings indicated that the compound could reduce oxidative stress markers and improve neuronal survival rates under conditions mimicking Alzheimer's disease. These results highlight its potential as a therapeutic candidate for neurodegenerative disorders .
Data Tables
Q & A
Basic Research Questions
Q. What are the established protocols for synthesizing 3-Ethyl-d5-adenine with isotopic purity ≥98%?
Methodological Answer: Synthesis typically involves deuterium exchange or incorporation during precursor synthesis. For example, deuterated ethyl groups can be introduced via catalytic deuteration of alkenes or alkyl halides using deuterium gas (D₂) in the presence of palladium catalysts . Isotopic purity is verified using liquid chromatography-mass spectrometry (LC-MS) coupled with nuclear magnetic resonance (NMR) to confirm deuterium incorporation at the C-3 ethyl position. Contamination risks arise from incomplete deuteration or isotopic exchange during purification; thus, inert atmospheres and deuterated solvents (e.g., D₂O, CDCl₃) are critical .
Q. How do researchers validate the structural integrity of this compound post-synthesis?
Methodological Answer: Structural validation requires multi-modal characterization:
- NMR : Compare protonated (¹H) and deuterated (²H) spectra to confirm deuterium substitution. Absence of proton signals at the C-3 ethyl group confirms isotopic labeling .
- High-Resolution Mass Spectrometry (HRMS) : Verify molecular ion peaks (e.g., [M+H]⁺) and isotopic distribution patterns to distinguish d5 from d4 or d6 analogs .
- Infrared Spectroscopy (IR) : Detect vibrational shifts in C-D bonds (2050–2300 cm⁻¹) versus C-H bonds (2800–3000 cm⁻¹) .
Q. What analytical techniques are most reliable for quantifying this compound in biological matrices?
Methodological Answer: Liquid chromatography-tandem mass spectrometry (LC-MS/MS) with stable isotope dilution is the gold standard. Key considerations:
- Internal Standards : Use isotopically labeled analogs (e.g., ¹³C or ¹⁵N variants) to correct for matrix effects .
- Extraction Efficiency : Optimize solid-phase extraction (SPE) protocols to minimize deuterium loss during sample preparation .
- Calibration Curves : Validate linearity across physiologically relevant concentrations (e.g., 0.1–100 ng/mL) with R² > 0.99 .
Advanced Research Questions
Q. How do deuterium kinetic isotope effects (KIEs) influence the metabolic stability of this compound in vivo?
Methodological Answer: KIEs arise from deuterium’s higher mass, slowing enzymatic cleavage of C-D bonds. To study this:
- In Vitro Assays : Incubate this compound with liver microsomes and quantify metabolites via LC-MS/MS. Compare half-lives (t₁/₂) with non-deuterated analogs .
- Computational Modeling : Use density functional theory (DFT) to predict bond dissociation energies (BDEs) and correlate with experimental degradation rates .
- Contradictions : Some studies report reduced metabolic clearance, while others note minimal effects due to competing metabolic pathways (e.g., glucuronidation). Resolve by profiling enzyme-specific interactions (e.g., CYP450 isoforms) .
Q. What strategies resolve spectral overlap when analyzing this compound in complex mixtures?
Methodological Answer:
- 2D-NMR : Use heteronuclear single-quantum coherence (HSQC) to separate overlapping signals in crowded spectra .
- Chromatographic Optimization : Adjust mobile phase pH or column chemistry (e.g., HILIC vs. reverse-phase) to improve separation .
- Data Deconvolution : Apply multivariate algorithms (e.g., MCR-ALS) to untangle co-eluting peaks in LC-MS datasets .
Q. How does isotopic labeling affect the crystallographic properties of this compound?
Methodological Answer: Deuterium’s neutron scattering cross-section enhances resolution in neutron diffraction studies. Methodological steps:
- Crystal Growth : Co-crystallize with heavy-atom derivatives (e.g., bromine) to improve phasing .
- Data Collection : Use neutron sources (e.g., Oak Ridge National Laboratory’s SNS) to capture deuterium positions, which are often invisible in X-ray diffraction .
- Contradictions : Some studies report altered lattice parameters due to isotopic substitution; resolve by comparing unit cell dimensions across protonated and deuterated crystals .
Q. Methodological Design and Data Contradictions
Q. How to design a controlled study comparing this compound’s pharmacokinetics across species?
Methodological Answer:
- Dosing Regimens : Administer equimolar doses to rodents and non-rodents, adjusting for metabolic rate differences (e.g., allometric scaling) .
- Sampling Protocol : Collect plasma/tissue samples at staggered intervals (e.g., 0.5, 1, 2, 4, 8 hours) to capture absorption/distribution phases .
- Statistical Analysis : Use mixed-effects modeling to account for interspecies variability and identify outliers .
Q. What experimental controls mitigate isotopic exchange in long-term stability studies?
Methodological Answer:
- Storage Conditions : Use amber vials under argon at −80°C to prevent light-/oxygen-induced degradation .
- Deuterium Retention Assays : Periodically analyze samples via LC-MS to track deuterium loss. Include non-deuterated controls to distinguish exchange from degradation .
- pH Monitoring : Maintain neutral pH buffers to minimize acid/base-catalyzed isotopic scrambling .
Q. Data Interpretation Challenges
Q. How to distinguish between deuterium loss due to metabolism vs. analytical artifacts?
Methodological Answer:
- Tracer Studies : Co-administer ³H-labeled analogs to track metabolic fate independently of deuterium .
- Artifact Controls : Spike samples with deuterated internal standards pre- and post-extraction to quantify procedural losses .
- High-Resolution MS/MS : Fragment ions containing deuterium (e.g., m/z shifts) to confirm isotopic retention in metabolites .
Q. What statistical approaches reconcile conflicting data on this compound’s enzyme inhibition potency?
Methodological Answer:
- Meta-Analysis : Pool data from multiple studies using random-effects models to account for heterogeneity .
- Sensitivity Analysis : Test whether outliers or assay conditions (e.g., substrate concentration) explain discrepancies .
- Enzyme Kinetics : Re-measure inhibition constants (Kᵢ) under standardized conditions (e.g., IC₅₀ at fixed ATP levels) .
Properties
IUPAC Name |
3-(1,1,2,2,2-pentadeuterioethyl)-7H-purin-6-imine | |
---|---|---|
Source | PubChem | |
URL | https://pubchem.ncbi.nlm.nih.gov | |
Description | Data deposited in or computed by PubChem | |
InChI |
InChI=1S/C7H9N5/c1-2-12-4-11-6(8)5-7(12)10-3-9-5/h3-4,8H,2H2,1H3,(H,9,10)/i1D3,2D2 | |
Source | PubChem | |
URL | https://pubchem.ncbi.nlm.nih.gov | |
Description | Data deposited in or computed by PubChem | |
InChI Key |
XAZTXNQITCBSCJ-ZBJDZAJPSA-N | |
Source | PubChem | |
URL | https://pubchem.ncbi.nlm.nih.gov | |
Description | Data deposited in or computed by PubChem | |
Canonical SMILES |
CCN1C=NC(=N)C2=C1N=CN2 | |
Source | PubChem | |
URL | https://pubchem.ncbi.nlm.nih.gov | |
Description | Data deposited in or computed by PubChem | |
Isomeric SMILES |
[2H]C([2H])([2H])C([2H])([2H])N1C=NC(=N)C2=C1N=CN2 | |
Source | PubChem | |
URL | https://pubchem.ncbi.nlm.nih.gov | |
Description | Data deposited in or computed by PubChem | |
Molecular Formula |
C7H9N5 | |
Source | PubChem | |
URL | https://pubchem.ncbi.nlm.nih.gov | |
Description | Data deposited in or computed by PubChem | |
DSSTOX Substance ID |
DTXSID80661951 | |
Record name | 3-(~2~H_5_)Ethyl-3H-purin-6-amine | |
Source | EPA DSSTox | |
URL | https://comptox.epa.gov/dashboard/DTXSID80661951 | |
Description | DSSTox provides a high quality public chemistry resource for supporting improved predictive toxicology. | |
Molecular Weight |
168.21 g/mol | |
Source | PubChem | |
URL | https://pubchem.ncbi.nlm.nih.gov | |
Description | Data deposited in or computed by PubChem | |
CAS No. |
147028-85-1 | |
Record name | 3-(~2~H_5_)Ethyl-3H-purin-6-amine | |
Source | EPA DSSTox | |
URL | https://comptox.epa.gov/dashboard/DTXSID80661951 | |
Description | DSSTox provides a high quality public chemistry resource for supporting improved predictive toxicology. | |
Disclaimer and Information on In-Vitro Research Products
Please be aware that all articles and product information presented on BenchChem are intended solely for informational purposes. The products available for purchase on BenchChem are specifically designed for in-vitro studies, which are conducted outside of living organisms. In-vitro studies, derived from the Latin term "in glass," involve experiments performed in controlled laboratory settings using cells or tissues. It is important to note that these products are not categorized as medicines or drugs, and they have not received approval from the FDA for the prevention, treatment, or cure of any medical condition, ailment, or disease. We must emphasize that any form of bodily introduction of these products into humans or animals is strictly prohibited by law. It is essential to adhere to these guidelines to ensure compliance with legal and ethical standards in research and experimentation.