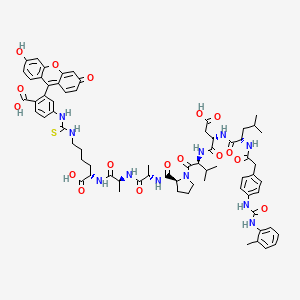
Ldv fitc
- Click on QUICK INQUIRY to receive a quote from our team of experts.
- With the quality product at a COMPETITIVE price, you can focus more on your research.
Overview
Description
LDV FITC is a fluorescent ligand that binds to the α4β1 integrin (VLA-4) with high affinity . It is used to detect VLA-4 affinity and conformational changes .
Molecular Structure Analysis
The molecular weight of LDV FITC is 1368.54 . Its formula is C69H81N11O17S . The sequence is LDVPAAK, with modifications: Leu-1 N-2- [4- [ [ [ (2-methylphenyl)amino]carbonyl]amino]phenyl]acetyl-, Lys-6 N-FITC conjugate .Physical And Chemical Properties Analysis
LDV FITC is soluble to 1 mg/ml in PBS (pH 7.4) . It should be stored at -20°C .Scientific Research Applications
Cell Adhesion Studies
LDV FITC is used in cell adhesion studies to detect VLA-4 affinity and conformational changes . It helps in understanding how cells interact with each other and their environment, which is crucial in many biological processes including tissue development and immune responses .
Cancer Research
In cancer research, LDV FITC has been used to study prostate cancer. A study synthesized a novel fluorescein isothiocyanate (FITC)-labeled prostate-specific membrane antigen (PSMA) ligand (PSMA-FITC) and evaluated its application value in targeted fluorescence imaging of PSMA-positive prostate cancer .
Immunology
LDV FITC is used in immunology to study the role of integrins in immune responses. For example, a study used LDV FITC to investigate the role of the α4β1 integrin in chronic lymphocytic leukemia .
Cell Biology
In cell biology, LDV FITC is used to study the role of integrins in cell adhesion, migration, and other cellular processes . It helps in understanding how cells interact with their environment and respond to various signals .
Pharmacology
LDV FITC can be used in pharmacology to study the interactions between drugs and integrins . This can help in the development of new drugs that target integrins.
Biochemistry
In biochemistry, LDV FITC can be used to study the biochemical properties of integrins and their ligands . This can provide insights into the molecular mechanisms underlying integrin function .
Molecular Biology
In molecular biology, LDV FITC can be used to study the role of integrins at the molecular level . This can help in understanding how integrins contribute to various cellular processes at the molecular level .
Mechanism of Action
Target of Action
LDV-FITC is a fluorescent peptide that is a FITC-conjugated LDV peptide . The primary target of LDV-FITC is the α4β1 integrin , also known as VLA-4 . Integrins are transmembrane receptors that facilitate cell-extracellular matrix adhesion. The α4β1 integrin plays a crucial role in cell migration and immune response, making it a significant target for LDV-FITC.
Mode of Action
LDV-FITC binds to the α4β1 integrin with high affinity . The binding affinity is measured with Kd values of 0.3 nM and 12 nM for binding to U937 cells in the presence and absence of Mn2+ respectively . This interaction allows LDV-FITC to influence the function of the α4β1 integrin, affecting cellular processes such as adhesion and migration.
Biochemical Pathways
The up-regulation of soluble LDV peptide or vascular cell adhesion molecule-1 (VCAM-1) binding by stimuli was critically dependent on activation of phospholipase C (PLC), inositol 1,4,5-triphosphate receptors, increased intracellular calcium, influx of extracellular calcium, and calmodulin . This suggests that these signaling pathways are required for α4 integrins to assume a high-affinity conformation.
Result of Action
The binding of LDV-FITC to the α4β1 integrin results in changes to the integrin’s function, affecting cellular processes such as adhesion and migration . By binding to the α4β1 integrin, LDV-FITC can influence these processes, potentially leading to changes in cell behavior.
Safety and Hazards
The safety data sheet of LDV FITC suggests using safety goggles with side-shields, protective gloves, and impervious clothing for handling . It also recommends using a suitable respirator . LDV FITC should be kept away from drains, water courses, or the soil .
Relevant Papers The relevant papers retrieved include studies on the affinity regulation of alpha 4-integrin , the use of conformational mAb as a tool for integrin ligand discovery , and the FRET detection of cellular α4-integrin conformational activation .
properties
IUPAC Name |
4-[[(5S)-5-carboxy-5-[[(2S)-2-[[(2S)-2-[[(2S)-1-[(2S)-2-[[(2S)-3-carboxy-2-[[(2S)-4-methyl-2-[[2-[4-[(2-methylphenyl)carbamoylamino]phenyl]acetyl]amino]pentanoyl]amino]propanoyl]amino]-3-methylbutanoyl]pyrrolidine-2-carbonyl]amino]propanoyl]amino]propanoyl]amino]pentyl]carbamothioylamino]-2-(3-hydroxy-6-oxoxanthen-9-yl)benzoic acid |
Source
|
---|---|---|
Source | PubChem | |
URL | https://pubchem.ncbi.nlm.nih.gov | |
Description | Data deposited in or computed by PubChem | |
InChI |
InChI=1S/C69H81N11O17S/c1-35(2)29-51(75-56(83)30-40-17-19-41(20-18-40)73-68(96)78-49-14-9-8-13-37(49)5)62(88)77-52(34-57(84)85)63(89)79-59(36(3)4)65(91)80-28-12-16-53(80)64(90)72-38(6)60(86)71-39(7)61(87)76-50(67(94)95)15-10-11-27-70-69(98)74-42-21-24-45(66(92)93)48(31-42)58-46-25-22-43(81)32-54(46)97-55-33-44(82)23-26-47(55)58/h8-9,13-14,17-26,31-33,35-36,38-39,50-53,59,81H,10-12,15-16,27-30,34H2,1-7H3,(H,71,86)(H,72,90)(H,75,83)(H,76,87)(H,77,88)(H,79,89)(H,84,85)(H,92,93)(H,94,95)(H2,70,74,98)(H2,73,78,96)/t38-,39-,50-,51-,52-,53-,59-/m0/s1 |
Source
|
Source | PubChem | |
URL | https://pubchem.ncbi.nlm.nih.gov | |
Description | Data deposited in or computed by PubChem | |
InChI Key |
PAAUNHNWVWSJKI-CBRXRBRLSA-N |
Source
|
Source | PubChem | |
URL | https://pubchem.ncbi.nlm.nih.gov | |
Description | Data deposited in or computed by PubChem | |
Canonical SMILES |
CC1=CC=CC=C1NC(=O)NC2=CC=C(C=C2)CC(=O)NC(CC(C)C)C(=O)NC(CC(=O)O)C(=O)NC(C(C)C)C(=O)N3CCCC3C(=O)NC(C)C(=O)NC(C)C(=O)NC(CCCCNC(=S)NC4=CC(=C(C=C4)C(=O)O)C5=C6C=CC(=O)C=C6OC7=C5C=CC(=C7)O)C(=O)O |
Source
|
Source | PubChem | |
URL | https://pubchem.ncbi.nlm.nih.gov | |
Description | Data deposited in or computed by PubChem | |
Isomeric SMILES |
CC1=CC=CC=C1NC(=O)NC2=CC=C(C=C2)CC(=O)N[C@@H](CC(C)C)C(=O)N[C@@H](CC(=O)O)C(=O)N[C@@H](C(C)C)C(=O)N3CCC[C@H]3C(=O)N[C@@H](C)C(=O)N[C@@H](C)C(=O)N[C@@H](CCCCNC(=S)NC4=CC(=C(C=C4)C(=O)O)C5=C6C=CC(=O)C=C6OC7=C5C=CC(=C7)O)C(=O)O |
Source
|
Source | PubChem | |
URL | https://pubchem.ncbi.nlm.nih.gov | |
Description | Data deposited in or computed by PubChem | |
Molecular Formula |
C69H81N11O17S |
Source
|
Source | PubChem | |
URL | https://pubchem.ncbi.nlm.nih.gov | |
Description | Data deposited in or computed by PubChem | |
Molecular Weight |
1368.5 g/mol |
Source
|
Source | PubChem | |
URL | https://pubchem.ncbi.nlm.nih.gov | |
Description | Data deposited in or computed by PubChem | |
Disclaimer and Information on In-Vitro Research Products
Please be aware that all articles and product information presented on BenchChem are intended solely for informational purposes. The products available for purchase on BenchChem are specifically designed for in-vitro studies, which are conducted outside of living organisms. In-vitro studies, derived from the Latin term "in glass," involve experiments performed in controlled laboratory settings using cells or tissues. It is important to note that these products are not categorized as medicines or drugs, and they have not received approval from the FDA for the prevention, treatment, or cure of any medical condition, ailment, or disease. We must emphasize that any form of bodily introduction of these products into humans or animals is strictly prohibited by law. It is essential to adhere to these guidelines to ensure compliance with legal and ethical standards in research and experimentation.