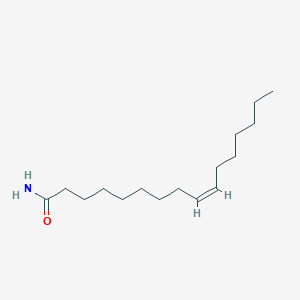
Palmitoleamide
- Click on QUICK INQUIRY to receive a quote from our team of experts.
- With the quality product at a COMPETITIVE price, you can focus more on your research.
Overview
Description
Mechanism of Action
Target of Action
The primary target of Palmitoleamide is proposed to be the peroxisome proliferator-activated receptor alpha (PPAR-α) . PPAR-α is a nuclear receptor that plays a crucial role in the regulation of lipid metabolism and inflammation . In addition to PPAR-α, this compound also has affinity to cannabinoid-like G-coupled receptors GPR55 and GPR119 .
Biochemical Analysis
Biochemical Properties
Palmitoleamide plays a significant role in biochemical reactions, particularly in the nervous system. It is involved in signaling pathways and interacts with various enzymes, proteins, and other biomolecules. One of the key interactions is with fatty acid amide hydrolase (FAAH), an enzyme responsible for the hydrolysis of fatty acid amides . This compound also interacts with peroxisome proliferator-activated receptor alpha (PPAR-α), a nuclear receptor that regulates gene expression related to lipid metabolism . These interactions highlight the compound’s role in modulating biochemical pathways and influencing cellular functions.
Cellular Effects
This compound exerts various effects on different cell types and cellular processes. It has been shown to influence cell signaling pathways, gene expression, and cellular metabolism. For instance, this compound can modulate the activity of PPAR-α, leading to changes in the expression of genes involved in lipid metabolism and inflammation . Additionally, it affects the function of mast cells, astrocytes, and microglia, which are crucial for immune responses and neuroinflammation . These cellular effects underscore the compound’s potential therapeutic applications in managing inflammatory and metabolic disorders.
Molecular Mechanism
The molecular mechanism of this compound involves its interaction with specific receptors and enzymes. This compound binds to PPAR-α, activating this receptor and leading to the transcription of target genes involved in lipid metabolism and anti-inflammatory responses . It also inhibits FAAH, resulting in increased levels of other fatty acid amides that contribute to its overall biological effects . These molecular interactions highlight the compound’s multifaceted role in regulating cellular processes and maintaining homeostasis.
Temporal Effects in Laboratory Settings
In laboratory settings, the effects of this compound can vary over time. Studies have shown that the compound is relatively stable and can exert long-term effects on cellular function. For example, prolonged exposure to this compound has been associated with sustained anti-inflammatory and neuroprotective effects . The stability and degradation of this compound can be influenced by factors such as temperature, pH, and the presence of specific enzymes . Understanding these temporal effects is crucial for optimizing its use in experimental and therapeutic applications.
Dosage Effects in Animal Models
The effects of this compound in animal models are dose-dependent. At lower doses, the compound has been shown to exert beneficial effects, such as reducing inflammation and pain . At higher doses, this compound may exhibit toxic or adverse effects, including alterations in liver function and metabolic disturbances . These findings highlight the importance of determining the optimal dosage for therapeutic applications to maximize benefits while minimizing potential risks.
Metabolic Pathways
This compound is involved in several metabolic pathways, including those related to fatty acid metabolism. It is synthesized from palmitoleic acid through the action of specific enzymes and can be degraded by FAAH . The compound also influences metabolic flux and metabolite levels by modulating the activity of PPAR-α and other metabolic regulators . These metabolic pathways underscore the compound’s role in maintaining cellular energy balance and regulating lipid homeostasis.
Transport and Distribution
Within cells and tissues, this compound is transported and distributed through interactions with specific transporters and binding proteins. It is produced from a glycerophospholipid precursor in the cell membrane and can be degraded by amidases located in the cell membrane and lysosome . The compound’s localization and accumulation are influenced by its interactions with these transporters and enzymes, affecting its overall bioavailability and biological activity.
Subcellular Localization
This compound’s subcellular localization is determined by various targeting signals and post-translational modifications. The compound is primarily localized in the cell membrane and lysosome, where it interacts with specific enzymes and receptors . These interactions are crucial for its activity and function, as they determine the compound’s accessibility to its molecular targets and its ability to modulate cellular processes.
Preparation Methods
Synthetic Routes and Reaction Conditions
Palmitoleamide can be synthesized through the formal condensation of palmitoleic acid with ammonia . The reaction typically involves the use of a catalyst to facilitate the condensation process. The reaction conditions include maintaining a specific temperature and pressure to ensure the efficient formation of this compound.
Industrial Production Methods
Industrial production of this compound involves the extraction of palmitoleic acid from natural sources such as fish oil and certain plant oils. The extracted palmitoleic acid is then subjected to the condensation reaction with ammonia under controlled conditions to produce this compound. The process may involve purification steps to obtain high-purity this compound suitable for various applications.
Chemical Reactions Analysis
Types of Reactions
Palmitoleamide undergoes various chemical reactions, including:
Oxidation: this compound can be oxidized to form corresponding oxides.
Reduction: It can be reduced to form saturated amides.
Substitution: this compound can undergo substitution reactions where the amide group is replaced by other functional groups.
Common Reagents and Conditions
Oxidation: Common oxidizing agents such as potassium permanganate or hydrogen peroxide can be used under acidic or basic conditions.
Reduction: Reducing agents like lithium aluminum hydride or sodium borohydride are commonly used.
Substitution: Various nucleophiles can be used in substitution reactions, often under mild conditions to prevent degradation of the compound.
Major Products
Oxidation: Oxidized derivatives of this compound.
Reduction: Saturated amides.
Substitution: Substituted amides with different functional groups.
Scientific Research Applications
Palmitoleamide has a wide range of scientific research applications, including:
Chemistry: Used as a model compound in studies of fatty acid amides and their chemical properties.
Biology: Investigated for its role as a metabolite in various biological systems.
Medicine: Explored for its potential therapeutic effects, including anti-inflammatory and analgesic properties.
Industry: Utilized in the production of bio-based materials and as a precursor for the synthesis of other bioactive compounds.
Comparison with Similar Compounds
Similar Compounds
Palmitoylethanolamide: An endogenous fatty acid amide with anti-inflammatory and analgesic properties.
Oleamide: Another fatty acid amide known for its sleep-inducing effects.
Anandamide: An endocannabinoid involved in pain modulation and inflammation.
Uniqueness
Palmitoleamide is unique due to its specific structure and the presence of a double bond in the fatty acid chain. This structural feature distinguishes it from other similar compounds and contributes to its unique biological activities and chemical properties.
Properties
IUPAC Name |
(Z)-hexadec-9-enamide |
Source
|
---|---|---|
Source | PubChem | |
URL | https://pubchem.ncbi.nlm.nih.gov | |
Description | Data deposited in or computed by PubChem | |
InChI |
InChI=1S/C16H31NO/c1-2-3-4-5-6-7-8-9-10-11-12-13-14-15-16(17)18/h7-8H,2-6,9-15H2,1H3,(H2,17,18)/b8-7- |
Source
|
Source | PubChem | |
URL | https://pubchem.ncbi.nlm.nih.gov | |
Description | Data deposited in or computed by PubChem | |
InChI Key |
YRPQTVNCCVPGFA-FPLPWBNLSA-N |
Source
|
Source | PubChem | |
URL | https://pubchem.ncbi.nlm.nih.gov | |
Description | Data deposited in or computed by PubChem | |
Canonical SMILES |
CCCCCCC=CCCCCCCCC(=O)N |
Source
|
Source | PubChem | |
URL | https://pubchem.ncbi.nlm.nih.gov | |
Description | Data deposited in or computed by PubChem | |
Isomeric SMILES |
CCCCCC/C=C\CCCCCCCC(=O)N |
Source
|
Source | PubChem | |
URL | https://pubchem.ncbi.nlm.nih.gov | |
Description | Data deposited in or computed by PubChem | |
Molecular Formula |
C16H31NO |
Source
|
Source | PubChem | |
URL | https://pubchem.ncbi.nlm.nih.gov | |
Description | Data deposited in or computed by PubChem | |
Molecular Weight |
253.42 g/mol |
Source
|
Source | PubChem | |
URL | https://pubchem.ncbi.nlm.nih.gov | |
Description | Data deposited in or computed by PubChem | |
Disclaimer and Information on In-Vitro Research Products
Please be aware that all articles and product information presented on BenchChem are intended solely for informational purposes. The products available for purchase on BenchChem are specifically designed for in-vitro studies, which are conducted outside of living organisms. In-vitro studies, derived from the Latin term "in glass," involve experiments performed in controlled laboratory settings using cells or tissues. It is important to note that these products are not categorized as medicines or drugs, and they have not received approval from the FDA for the prevention, treatment, or cure of any medical condition, ailment, or disease. We must emphasize that any form of bodily introduction of these products into humans or animals is strictly prohibited by law. It is essential to adhere to these guidelines to ensure compliance with legal and ethical standards in research and experimentation.