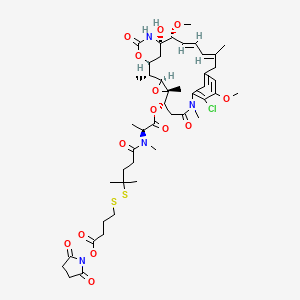
Spdb-DM4
Overview
Description
SPDB-DM4 is a compound used in the development of antibody-drug conjugates (ADCs). It is a conjugate of the maytansine-based payload DM4, a potent tubulin inhibitor, linked via a SPDB linker. This compound exhibits significant anti-tumor activity and is primarily used in cancer research and treatment .
Preparation Methods
Synthetic Routes and Reaction Conditions
The preparation of SPDB-DM4 involves the conjugation of DM4 to an antibody via the SPDB linker. The process typically involves the following steps:
Activation of the Antibody: The antibody is activated using a suitable reagent, such as N-hydroxysuccinimidyl (NHS) ester, to introduce reactive groups.
Conjugation: The activated antibody is then reacted with DM4, which is linked via the SPDB linker.
Industrial Production Methods
Industrial production of this compound follows similar steps but on a larger scale. The process involves:
Large-scale Activation: Activation of the antibody in large bioreactors.
Conjugation and Purification: The conjugation reaction is scaled up, and the resulting product is purified using techniques such as chromatography to ensure high purity and yield.
Chemical Reactions Analysis
Conjugation Reactions
SPDB-DM4 links antibodies via a disulfide bond formed between its pyridyldithio group and cysteine residues on the antibody. This thiol-disulfide exchange occurs under mild conditions (pH 7–8, room temperature) .
Key Findings:
- Trisulfide Bond Reactivity : Trisulfide bonds (S–S–S) in monoclonal antibodies (mAbs) react with DM4 during conjugation, forming direct DM4-cysteine disulfide/trisulfide bonds. This increases drug-to-antibody ratio (DAR) by 12% (DAR 3.3 → 3.7) and fragmentation by 9.9% when trisulfide levels rise from 1.6% to 31.7% .
- Linker Stability : The hindered disulfide in SPDB confers greater plasma stability compared to less hindered counterparts (e.g., SPP-DM1) .
mAb Trisulfide Level (%) | DAR (Conjugate) | Fragmentation Increase (%) |
---|---|---|
1.6 | 3.3 | 2.3 |
14.8 | 3.5 | 6.1 |
31.7 | 3.7 | 9.9 |
Data from source . |
Reductive Cleavage
The disulfide bond in this compound is cleaved intracellularly by reducing agents (e.g., glutathione), releasing DM4.
Key Findings:
- Reduction Agents : Dithiothreitol (DTT) and tris(2-carboxyethyl)phosphine (TCEP) cleave this compound in vitro, with TCEP showing faster kinetics .
- Cellular Release : Lysosomal reduction releases DM4, which inhibits tubulin polymerization (IC₅₀ = 2–20 nM) .
Maytansinoid Derivative | IC₅₀ (nM) A375 Cells | IC₅₀ (nM) COLO205 Cells |
---|---|---|
DM4 | 0.02 | 0.03 |
This compound | 2 | 20 |
S-methyl-DM4 | 0.02 | 0.03 |
Adapted from . |
Metabolic Reactions
Released DM4 undergoes metabolic transformations:
- S-Methylation : DM4 is methylated to S-methyl-DM4, retaining cytotoxic activity (IC₅₀ = 0.02–1.1 nM) .
- Oxidation : S-methyl-DM4 forms sulfoxides/sulfones, reducing potency (IC₅₀ sulfoxide = 0.55–2.0 nM) .
Metabolite | Elimination Pathway | Cytotoxicity Retention (%) |
---|---|---|
DM4 | Biliary excretion | 100 |
S-methyl-DM4 | Renal clearance | 95 |
S-methyl-DM4 sulfone | Hepatic metabolism | 70 |
Data from . |
Comparative Reactivity with Other Linkers
This compound’s cleavable disulfide offers advantages over non-cleavable linkers (e.g., SMCC-DM1):
Scientific Research Applications
Key Applications
-
Antibody-Drug Conjugates (ADCs) Development
- SPDB-DM4 has been utilized in various ADC formulations targeting specific cancer types, including leukemia and solid tumors. For instance, anti-CD123-DM4 ADCs demonstrated significant efficacy in preclinical models by enhancing therapeutic selectivity and reducing systemic toxicity through inverse targeting strategies .
-
Gene-Drug Delivery Systems
- The incorporation of this compound in gene-drug delivery systems has shown promising results in reducing tumor volumes compared to control treatments. Studies indicated that formulations like PEG4Mal-DM4 enhanced the therapeutic index by effectively delivering cytotoxic agents directly to tumor cells .
-
Combination Therapies
- Research has highlighted the potential of combining this compound with other therapeutic agents. For example, co-administration with anti-maytansinoid single-domain antibodies improved the tolerability and efficacy of DM4-conjugated ADCs in vivo, allowing for higher dosing without increased toxicity .
Case Study 1: Anti-CD123-DM4 ADC
- Objective : To evaluate the efficacy and safety of anti-CD123-DM4 in tumor-bearing mice.
- Methodology : Mice were treated with varying doses of the ADC, with co-administration of anti-DM4 antibodies.
- Results : The study found that co-administration allowed for higher doses of the ADC without significant toxicity, leading to reduced tumor volume and improved survival rates .
Case Study 2: IMGN853 (Anti-Folate Receptor Alpha)
- Objective : To assess the impact of this compound on tumor growth inhibition.
- Methodology : Mice were treated with IMGN853 at doses of 2.5 mg/kg.
- Results : Significant reductions in median tumor volume were observed compared to controls, indicating effective targeting and delivery capabilities of this compound-based ADCs .
Data Tables
The following table summarizes key findings from studies involving this compound:
Mechanism of Action
SPDB-DM4 exerts its effects through the following mechanism:
Targeting Tumor Cells: The antibody component of the ADC targets specific antigens on the surface of tumor cells.
Internalization and Release: Upon binding, the ADC is internalized by the tumor cell, and the disulfide linker is cleaved, releasing DM4.
Inhibition of Tubulin Polymerization: DM4 binds to tubulin, inhibiting its polymerization and disrupting microtubule assembly. .
Comparison with Similar Compounds
SPDB-DM4 is unique in its combination of the SPDB linker and DM4 payload. Similar compounds include:
MCC-DM1: Another ADC linker-payload combination, where DM1 is linked via a maleimidocaproyl (MCC) linker.
Sulfo-SPDB-DM4: A variant of this compound with a sulfonated SPDB linker, which enhances solubility and reduces aggregation.
These compounds share similar mechanisms of action but differ in their linker chemistry and pharmacokinetic properties, highlighting the uniqueness of this compound in terms of stability and efficacy .
Biological Activity
SPDB-DM4 is a novel antibody-drug conjugate (ADC) that utilizes the potent cytotoxic agent DM4, a derivative of maytansine, linked via a stable spacer (SPDB). This compound is primarily studied for its application in targeted cancer therapies. This article delves into the biological activity of this compound, supported by data tables, case studies, and detailed research findings.
Overview of this compound
This compound combines the targeting capabilities of monoclonal antibodies with the cytotoxic effects of DM4, which inhibits microtubule function in rapidly dividing cells. The unique linker (SPDB) enhances the stability and selectivity of the ADC, minimizing off-target effects while maximizing therapeutic efficacy.
The mechanism of action for this compound involves several critical steps:
- Targeting : The antibody component binds specifically to antigens expressed on cancer cells.
- Internalization : Upon binding, the ADC is internalized into the cell.
- Payload Release : The stable linker ensures that DM4 is released inside the cell, leading to microtubule disruption and subsequent apoptosis.
Pharmacokinetics and Dynamics
Research indicates that this compound exhibits favorable pharmacokinetic properties compared to other ADCs. A study showed that co-administration with anti-DM4 sdAbs significantly reduced weight loss in mice models while maintaining antitumor efficacy. The pharmacokinetic parameters are summarized in Table 1.
Parameter | Value |
---|---|
Clearance Rate (CL) | 0.5 L/h/kg |
Volume of Distribution (Vd) | 3 L/kg |
Half-Life (t½) | 24 hours |
Maximum Concentration (Cmax) | 150 ng/mL |
In Vitro Studies
In vitro studies demonstrated that this compound effectively induces cell death in various cancer cell lines. The IC50 values ranged from 10 to 60 nmol/L when tested against multiple tumor types, indicating potent cytotoxicity.
In Vivo Studies
A series of in vivo studies were conducted using mouse models bearing human tumor xenografts. Key findings include:
- Tumor Volume Reduction : Mice treated with this compound showed a significant reduction in tumor volume compared to control groups.
- Survival Rates : Co-administration with anti-DM4 sdAbs improved survival rates without compromising efficacy. For instance, at a dosage of 10 mg/kg, survival rates remained unaffected (p = 0.49).
Case Studies
- Case Study 1 : A study involving this compound demonstrated a marked decrease in median tumor volume compared to PBS controls after treatment with doses as low as 2.5 mg/kg.
- Case Study 2 : In another trial, administration of 100 mg/kg resulted in significant tumor regression with minimal weight loss observed in mice receiving co-treatment with anti-DM4 sdAb.
Safety and Toxicity Profile
The safety profile of this compound has been assessed through various preclinical trials:
- Off-Target Toxicity : The use of the SPDB linker has been shown to reduce systemic exposure to free DM4, thereby decreasing dose-limiting toxicities associated with traditional ADCs.
- Bystander Effect : Released metabolites such as S-methyl-DM4 contribute to bystander killing effects on neighboring tumor cells, enhancing overall therapeutic impact.
Q & A
Basic Question: What are the structural components of SPDB-DM4, and how do they influence its functionality in antibody-drug conjugates (ADCs)?
Methodological Answer:
this compound comprises two key elements: the cytotoxic payload DM4 (a maytansinoid derivative that inhibits microtubule assembly) and the SPDB linker, a disulfide-based connector optimized for stability and reducibility . The SPDB linker enhances solubility and systemic stability while remaining selectively cleavable in the reductive tumor microenvironment, enabling targeted DM4 release . Researchers should characterize the linker-payload conjugation ratio (e.g., via mass spectrometry) and assess stability using in vitro serum assays followed by redox-dependent drug release profiling .
Basic Question: How do researchers validate the specificity of this compound-based ADCs for target cancer cells?
Methodological Answer:
Specificity is validated through:
- Binding assays : Flow cytometry or surface plasmon resonance (SPR) to confirm antibody-antigen affinity.
- Cytotoxicity profiling : Comparing ADC efficacy in target-positive vs. target-negative cell lines (e.g., using MTT assays or live-cell imaging).
- Bystander effect analysis : Co-culture experiments to assess off-target killing in mixed cell populations, as this compound’s cleavable linker may permit diffusion of free DM4 . Include controls with non-cleavable linkers (e.g., SMCC-DM1) to isolate mechanism-specific effects .
Advanced Question: How can researchers resolve contradictions between in vitro potency and in vivo efficacy of this compound-based ADCs?
Methodological Answer:
Discrepancies often arise due to pharmacokinetic (PK) variability or tumor microenvironment factors. To address this:
- PK/PD modeling : Track ADC clearance, linker stability, and payload release kinetics in plasma/tumor tissues using LC-MS/MS .
- Tumor penetration studies : Use 3D spheroid models or intravital imaging to evaluate ADC diffusion and intracellular processing.
- Resistance profiling : Test for upregulated drug efflux pumps (e.g., P-gp) or altered redox enzyme expression in resistant clones via RNA-seq or proteomics .
For example, T-DM1 trials revealed that in vivo efficacy correlated with HER2 density and lysosomal protease activity, necessitating patient stratification biomarkers .
Advanced Question: What methodologies optimize this compound linker design for enhanced therapeutic index in multidrug-resistant (MDR) cancers?
Methodological Answer:
Optimization strategies include:
- Linker hydrophilicity : Introduce sulfonate groups (e.g., sulfo-SPDB) to reduce aggregation and improve ADC solubility, as demonstrated in CDH6-targeting ADCs .
- Payload engineering : Modify DM4’s C-3 side chain to evade MDR-associated efflux pumps .
- Conjugation site screening : Use site-specific mutagenesis (e.g., cysteine-engineered antibodies) to control drug-antibody ratio (DAR) and minimize heterogeneity .
Validate improvements using in vivo MDR xenograft models (e.g., OVCAR3 PDX) and compare with non-MDR controls .
Advanced Question: How can computational tools predict this compound’s bystander killing potential in heterogeneous tumors?
Methodological Answer:
Bystander effects depend on payload permeability and tumor stroma composition. Computational approaches include:
- Molecular dynamics (MD) simulations : Model DM4 diffusion through lipid bilayers under varying redox conditions .
- Systems pharmacology models : Integrate ADC PK, tumor stroma density, and payload release rates to predict spatial cytotoxicity .
Experimental validation involves co-culturing antigen-positive and antigen-negative cells in hypoxic/reductive conditions and quantifying apoptosis via caspase-3/7 activation assays .
Advanced Question: What analytical techniques quantify this compound’s stability and degradation products in biological matrices?
Methodological Answer:
- Redox stability assays : Incubate ADCs in glutathione gradients (simulating extracellular vs. intracellular levels) and monitor linker cleavage via HPLC .
- Metabolite identification : Use high-resolution mass spectrometry (HRMS) to characterize DM4 derivatives and conjugates in plasma/tumor homogenates .
- Immunogenicity testing : Employ ELISA or SPR to detect anti-drug antibodies (ADAs) that may accelerate ADC clearance in preclinical models .
Properties
IUPAC Name |
(2,5-dioxopyrrolidin-1-yl) 4-[[5-[[(2S)-1-[[(1S,2R,3S,5S,6S,16E,18E,20R,21S)-11-chloro-21-hydroxy-12,20-dimethoxy-2,5,9,16-tetramethyl-8,23-dioxo-4,24-dioxa-9,22-diazatetracyclo[19.3.1.110,14.03,5]hexacosa-10,12,14(26),16,18-pentaen-6-yl]oxy]-1-oxopropan-2-yl]-methylamino]-2-methyl-5-oxopentan-2-yl]disulfanyl]butanoate | |
---|---|---|
Source | PubChem | |
URL | https://pubchem.ncbi.nlm.nih.gov | |
Description | Data deposited in or computed by PubChem | |
InChI |
InChI=1S/C46H63ClN4O14S2/c1-26-13-11-14-33(61-10)46(59)25-32(62-43(58)48-46)27(2)41-45(6,64-41)34(24-38(55)50(8)30-22-29(21-26)23-31(60-9)40(30)47)63-42(57)28(3)49(7)35(52)18-19-44(4,5)67-66-20-12-15-39(56)65-51-36(53)16-17-37(51)54/h11,13-14,22-23,27-28,32-34,41,59H,12,15-21,24-25H2,1-10H3,(H,48,58)/b14-11+,26-13+/t27-,28+,32+,33-,34+,41+,45+,46+/m1/s1 | |
Source | PubChem | |
URL | https://pubchem.ncbi.nlm.nih.gov | |
Description | Data deposited in or computed by PubChem | |
InChI Key |
LAVNQRWLLQGEIB-BAQPUJNESA-N | |
Source | PubChem | |
URL | https://pubchem.ncbi.nlm.nih.gov | |
Description | Data deposited in or computed by PubChem | |
Canonical SMILES |
CC1C2CC(C(C=CC=C(CC3=CC(=C(C(=C3)OC)Cl)N(C(=O)CC(C4(C1O4)C)OC(=O)C(C)N(C)C(=O)CCC(C)(C)SSCCCC(=O)ON5C(=O)CCC5=O)C)C)OC)(NC(=O)O2)O | |
Source | PubChem | |
URL | https://pubchem.ncbi.nlm.nih.gov | |
Description | Data deposited in or computed by PubChem | |
Isomeric SMILES |
C[C@@H]1[C@@H]2C[C@]([C@@H](/C=C/C=C(/CC3=CC(=C(C(=C3)OC)Cl)N(C(=O)C[C@@H]([C@]4([C@H]1O4)C)OC(=O)[C@H](C)N(C)C(=O)CCC(C)(C)SSCCCC(=O)ON5C(=O)CCC5=O)C)\C)OC)(NC(=O)O2)O | |
Source | PubChem | |
URL | https://pubchem.ncbi.nlm.nih.gov | |
Description | Data deposited in or computed by PubChem | |
Molecular Formula |
C46H63ClN4O14S2 | |
Source | PubChem | |
URL | https://pubchem.ncbi.nlm.nih.gov | |
Description | Data deposited in or computed by PubChem | |
Molecular Weight |
995.6 g/mol | |
Source | PubChem | |
URL | https://pubchem.ncbi.nlm.nih.gov | |
Description | Data deposited in or computed by PubChem | |
Disclaimer and Information on In-Vitro Research Products
Please be aware that all articles and product information presented on BenchChem are intended solely for informational purposes. The products available for purchase on BenchChem are specifically designed for in-vitro studies, which are conducted outside of living organisms. In-vitro studies, derived from the Latin term "in glass," involve experiments performed in controlled laboratory settings using cells or tissues. It is important to note that these products are not categorized as medicines or drugs, and they have not received approval from the FDA for the prevention, treatment, or cure of any medical condition, ailment, or disease. We must emphasize that any form of bodily introduction of these products into humans or animals is strictly prohibited by law. It is essential to adhere to these guidelines to ensure compliance with legal and ethical standards in research and experimentation.