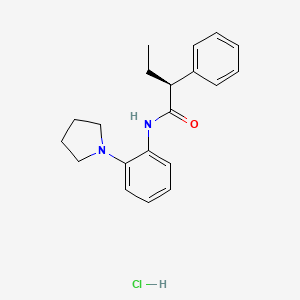
ML252
- Click on QUICK INQUIRY to receive a quote from our team of experts.
- With the quality product at a COMPETITIVE price, you can focus more on your research.
Overview
Description
ML252, also known as (S)-2-Phenyl-N-(2-(pyrrolidin-1-yl)phenyl)butanamide, is a potent and selective inhibitor of the voltage-gated potassium channel Kv7.2 (KCNQ2). This compound is brain-penetrant and exhibits significant selectivity for Kv7.2 over other potassium channels, making it a valuable tool in neuroscience research .
Mechanism of Action
Target of Action
The primary target of ML252 is the Kv7.2 voltage-gated potassium channels . These channels are critical regulators of neuronal excitability and are candidate targets for the development of antiseizure medications .
Mode of Action
This compound acts as a potent and selective inhibitor of Kv7.2 channels . It binds to a specific tryptophan residue in the pore of the Kv7.2 and Kv7.3 channels, which is also required for sensitivity to certain activators . This binding inhibits the function of the channels, modulating their role in neuronal excitability .
Biochemical Pathways
The inhibition of Kv7.2 channels by this compound affects the neuronal excitability, which is regulated by these channels
Pharmacokinetics
It is known that this compound is brain penetrant , suggesting it can cross the blood-brain barrier. This is crucial for its role in modulating neuronal excitability.
Result of Action
The inhibition of Kv7.2 channels by this compound increases neuronal excitability . This can have various effects at the molecular and cellular level, depending on the specific neuronal pathways involved.
Biochemical Analysis
Biochemical Properties
ML252 plays a crucial role in biochemical reactions, particularly as an inhibitor of KV7.2 voltage-gated potassium channels . It interacts with these channels and inhibits their function, displaying a selectivity that is more than 40-fold for KV7.2 over KV7.1 channels . The nature of these interactions involves the binding of this compound to the channels, resulting in their inhibition .
Cellular Effects
This compound has profound effects on various types of cells and cellular processes. It influences cell function by inhibiting the KV7.2 voltage-gated potassium channels, which are critical regulators of neuronal excitability . This inhibition can impact cell signaling pathways, gene expression, and cellular metabolism .
Molecular Mechanism
The mechanism of action of this compound involves its binding interactions with KV7.2 voltage-gated potassium channels . It exerts its effects at the molecular level by inhibiting these channels, leading to changes in gene expression and cellular metabolism .
Metabolic Pathways
This compound is involved in metabolic pathways related to the function of KV7.2 voltage-gated potassium channels . It interacts with these channels and can potentially affect metabolic flux or metabolite levels .
Transport and Distribution
It is known to interact with KV7.2 voltage-gated potassium channels
Subcellular Localization
It is known to interact with KV7.2 voltage-gated potassium channels
Preparation Methods
Synthetic Routes and Reaction Conditions
The synthesis of ML252 involves several key steps:
Formation of the amide bond: The starting material, 2-phenylbutanoic acid, is reacted with 2-(pyrrolidin-1-yl)aniline in the presence of a coupling agent such as N,N’-dicyclohexylcarbodiimide (DCC) and 4-dimethylaminopyridine (DMAP) to form the amide bond.
Purification: The crude product is purified using column chromatography to obtain the desired compound with high purity.
Industrial Production Methods
While specific industrial production methods for this compound are not widely documented, the general approach would involve scaling up the synthetic route mentioned above. This would include optimizing reaction conditions, using larger reaction vessels, and employing industrial-scale purification techniques such as recrystallization or large-scale chromatography.
Chemical Reactions Analysis
Types of Reactions
ML252 primarily undergoes the following types of reactions:
Oxidation: this compound can be oxidized under specific conditions, although detailed oxidation pathways are not extensively documented.
Reduction: The compound can undergo reduction reactions, particularly at the amide functional group.
Substitution: this compound can participate in substitution reactions, especially at the aromatic rings.
Common Reagents and Conditions
Oxidation: Common oxidizing agents such as potassium permanganate or chromium trioxide can be used.
Reduction: Reducing agents like lithium aluminum hydride or sodium borohydride are typically employed.
Substitution: Electrophilic aromatic substitution can be carried out using reagents like bromine or nitric acid under controlled conditions.
Major Products
The major products formed from these reactions depend on the specific conditions and reagents used. For example, oxidation may yield carboxylic acids or ketones, while reduction may produce amines or alcohols.
Scientific Research Applications
ML252 has a wide range of scientific research applications:
Comparison with Similar Compounds
Similar Compounds
Retigabine: A Kv7 channel activator used in the treatment of epilepsy.
ML213: Another Kv7 channel inhibitor with a similar binding site as ML252.
ICA-069673: A Kv7 channel activator that targets the voltage sensor rather than the pore.
Uniqueness of this compound
This compound is unique due to its high selectivity for Kv7.2 channels over other potassium channels and its ability to penetrate the brain. This selectivity and brain penetration make it particularly useful for studying the physiological roles of Kv7.2 channels and for exploring potential therapeutic applications in neurological disorders .
Properties
IUPAC Name |
(2S)-2-phenyl-N-(2-pyrrolidin-1-ylphenyl)butanamide;hydrochloride |
Source
|
---|---|---|
Source | PubChem | |
URL | https://pubchem.ncbi.nlm.nih.gov | |
Description | Data deposited in or computed by PubChem | |
InChI |
InChI=1S/C20H24N2O.ClH/c1-2-17(16-10-4-3-5-11-16)20(23)21-18-12-6-7-13-19(18)22-14-8-9-15-22;/h3-7,10-13,17H,2,8-9,14-15H2,1H3,(H,21,23);1H/t17-;/m0./s1 |
Source
|
Source | PubChem | |
URL | https://pubchem.ncbi.nlm.nih.gov | |
Description | Data deposited in or computed by PubChem | |
InChI Key |
XBYCQOZNTLUEDX-LMOVPXPDSA-N |
Source
|
Source | PubChem | |
URL | https://pubchem.ncbi.nlm.nih.gov | |
Description | Data deposited in or computed by PubChem | |
Canonical SMILES |
CCC(C1=CC=CC=C1)C(=O)NC2=CC=CC=C2N3CCCC3.Cl |
Source
|
Source | PubChem | |
URL | https://pubchem.ncbi.nlm.nih.gov | |
Description | Data deposited in or computed by PubChem | |
Isomeric SMILES |
CC[C@@H](C1=CC=CC=C1)C(=O)NC2=CC=CC=C2N3CCCC3.Cl |
Source
|
Source | PubChem | |
URL | https://pubchem.ncbi.nlm.nih.gov | |
Description | Data deposited in or computed by PubChem | |
Molecular Formula |
C20H25ClN2O |
Source
|
Source | PubChem | |
URL | https://pubchem.ncbi.nlm.nih.gov | |
Description | Data deposited in or computed by PubChem | |
Molecular Weight |
344.9 g/mol |
Source
|
Source | PubChem | |
URL | https://pubchem.ncbi.nlm.nih.gov | |
Description | Data deposited in or computed by PubChem | |
Q1: What is the mechanism of action of ML252 and how does it impact neuronal excitability?
A: this compound functions as a pore-targeted inhibitor of Kv7.2/Kv7.3 voltage-gated potassium channels. [] These channels are crucial for regulating neuronal excitability. By binding to a specific tryptophan residue (W236 in Kv7.2 and W265 in Kv7.3) within the channel pore, this compound prevents the flow of potassium ions, leading to increased neuronal excitability. [] This interaction site within the pore overlaps with the binding site of certain Kv7 activators, like ML213, leading to competitive interactions between these compounds. []
Q2: How does the structure of this compound influence its activity as a Kv7.2 channel modulator?
A: Interestingly, minor structural modifications to this compound can drastically alter its activity profile on Kv7.2 channels. Replacing the ethyl group of this compound with a hydrogen atom converts the compound from a potent antagonist (inhibitor) to an agonist (activator). [] This highlights the critical role of even subtle structural features in determining the compound's interaction with the channel and its subsequent functional effects. This sensitivity to structural changes underscores the importance of structure-activity relationship (SAR) studies in understanding and optimizing the pharmacological properties of this compound and related compounds. []
Q3: What experimental evidence supports the in vivo activity of this compound on neuronal activity?
A: Studies utilizing transgenic zebrafish larvae expressing a genetically encoded calcium indicator (CaMPARI) provide compelling evidence for this compound's in vivo activity. [] These studies demonstrated that this compound effectively increases neuronal activity in living organisms, consistent with its inhibitory effects on Kv7 channels observed in vitro. [] Moreover, the in vivo experiments further validated the competitive interaction between this compound and the pore-targeted Kv7 activator ML213, as co-administration of ML213 effectively suppressed the this compound-induced increase in neuronal activity. [] This highlights the potential of utilizing this compound as a tool to study Kv7 channel function in vivo and screen for novel compounds targeting these channels.
Disclaimer and Information on In-Vitro Research Products
Please be aware that all articles and product information presented on BenchChem are intended solely for informational purposes. The products available for purchase on BenchChem are specifically designed for in-vitro studies, which are conducted outside of living organisms. In-vitro studies, derived from the Latin term "in glass," involve experiments performed in controlled laboratory settings using cells or tissues. It is important to note that these products are not categorized as medicines or drugs, and they have not received approval from the FDA for the prevention, treatment, or cure of any medical condition, ailment, or disease. We must emphasize that any form of bodily introduction of these products into humans or animals is strictly prohibited by law. It is essential to adhere to these guidelines to ensure compliance with legal and ethical standards in research and experimentation.