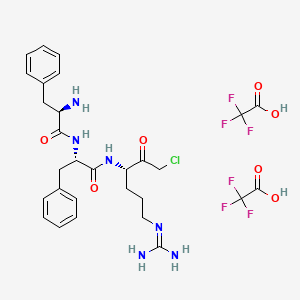
PPACKII (trifluoroacetate salt)
Overview
Description
Mechanism of Action
Target of Action
PPACKII (Trifluoroacetate Salt), also known as PPACK II (diTFA), is a potent and specific irreversible inhibitor of plasma and glandular kallikreins . Kallikreins are a class of secreted serine proteases that play a crucial role in various physiological processes .
Mode of Action
PPACKII interacts with its targets, the plasma and glandular kallikreins, in an irreversible manner . It inhibits the enzymatic activity of these kallikreins, preventing them from catalyzing the release of vasodepressor peptides or kinins from a plasma substrate .
Biochemical Pathways
The inhibition of kallikreins by PPACKII affects the biochemical pathways involving the release of vasodepressor peptides or kinins . These peptides play a significant role in blood pressure regulation, inflammation, and pain induction. By inhibiting kallikreins, PPACKII can potentially influence these physiological processes .
Result of Action
The primary result of PPACKII’s action is the inhibition of plasma and glandular kallikreins, leading to a decrease in the release of vasodepressor peptides or kinins . This can have various downstream effects, including potential impacts on blood pressure regulation, inflammation, and pain response .
Action Environment
The action, efficacy, and stability of PPACKII can be influenced by various environmental factors. For instance, the pH of the environment can affect the solubility and therefore the bioavailability of the compound . .
Biochemical Analysis
Biochemical Properties
PPACKII (trifluoroacetate salt) interacts with human tissue kallikreins (hKs), a class of secreted serine proteases . These enzymes are involved in the release of vasodepressor peptides or kinins from a plasma substrate . The interaction between PPACKII (trifluoroacetate salt) and these enzymes is irreversible, making it a potent inhibitor .
Cellular Effects
The trifluoroacetate salt has been found to have effects on various types of cells. For instance, it has been reported to inhibit the proliferation of osteoblasts and chondrocytes . It’s also indicated that trifluoroacetate is a weak peroxisome proliferator in rats .
Molecular Mechanism
PPACKII (trifluoroacetate salt) exerts its effects at the molecular level by inhibiting the activity of kallikreins . It prevents apolipoprotein proteolysis in dextran sulfate-precipitated human plasma LDL and inhibits serum atrial natriuretic peptide cleavage at micromolar concentrations . It has also been reported that PPACKII (trifluoroacetate salt) inactivates the amidolytic activity of native human Hageman Factor (Factor XII) at 5.3 μM .
Temporal Effects in Laboratory Settings
It’s recommended that the solution should be used up soon after reconstitution, indicating potential stability or degradation issues .
Dosage Effects in Animal Models
Oral repeated dose studies in rats have identified the liver as the target organ with mild liver hypertrophy as the lead effect .
Preparation Methods
Synthetic Routes and Reaction Conditions
The synthesis of PPACK II (diTFA) involves the reaction of specific amino acid derivatives under controlled conditions. The process typically includes the following steps:
Formation of the peptide backbone: The peptide backbone is synthesized using standard solid-phase peptide synthesis techniques.
Introduction of the trifluoroacetate group: The trifluoroacetate group is introduced through a reaction with trifluoroacetic acid under acidic conditions.
Purification: The final product is purified using chromatographic techniques to ensure high purity and yield.
Industrial Production Methods
Industrial production of PPACK II (diTFA) follows similar synthetic routes but on a larger scale. The process involves:
Bulk synthesis: Large-scale solid-phase peptide synthesis is employed to produce the peptide backbone.
Trifluoroacetate introduction: The trifluoroacetate group is introduced in large reactors under controlled conditions.
Purification and quality control: The product is purified using industrial-scale chromatography and subjected to rigorous quality control to ensure consistency and purity.
Chemical Reactions Analysis
Types of Reactions
PPACK II (diTFA) primarily undergoes inhibition reactions with kallikreins. It does not typically participate in oxidation, reduction, or substitution reactions due to its specific inhibitory function .
Common Reagents and Conditions
The inhibition reaction involves the following:
Reagents: PPACK II (diTFA) and kallikreins.
Conditions: The reaction occurs under physiological conditions, typically at a pH of 7.4 and a temperature of 37°C.
Major Products
The major product of the reaction is the inhibited kallikrein enzyme, which is rendered inactive by the irreversible binding of PPACK II (diTFA) .
Scientific Research Applications
PPACK II (diTFA) has several scientific research applications, including:
Biochemistry: Used to study the role of kallikreins in various physiological processes.
Pharmacology: Employed in the development of therapeutic agents targeting kallikreins.
Industry: Utilized in the production of diagnostic kits and assays for detecting kallikrein activity.
Comparison with Similar Compounds
Similar Compounds
PPACK: Another irreversible inhibitor of kallikreins, but without the trifluoroacetate group.
DIPF: Diisopropyl fluorophosphate, a general serine protease inhibitor.
Aprotinin: A reversible inhibitor of kallikreins and other serine proteases.
Uniqueness
PPACK II (diTFA) is unique due to its irreversible inhibition mechanism and enhanced water solubility and stability provided by the trifluoroacetate group . This makes it particularly useful in research and industrial applications where stability and solubility are critical.
Properties
IUPAC Name |
(2R)-2-amino-N-[(2S)-1-[[(3S)-1-chloro-6-(diaminomethylideneamino)-2-oxohexan-3-yl]amino]-1-oxo-3-phenylpropan-2-yl]-3-phenylpropanamide;2,2,2-trifluoroacetic acid | |
---|---|---|
Source | PubChem | |
URL | https://pubchem.ncbi.nlm.nih.gov | |
Description | Data deposited in or computed by PubChem | |
InChI |
InChI=1S/C25H33ClN6O3.2C2HF3O2/c26-16-22(33)20(12-7-13-30-25(28)29)31-24(35)21(15-18-10-5-2-6-11-18)32-23(34)19(27)14-17-8-3-1-4-9-17;2*3-2(4,5)1(6)7/h1-6,8-11,19-21H,7,12-16,27H2,(H,31,35)(H,32,34)(H4,28,29,30);2*(H,6,7)/t19-,20+,21+;;/m1../s1 | |
Source | PubChem | |
URL | https://pubchem.ncbi.nlm.nih.gov | |
Description | Data deposited in or computed by PubChem | |
InChI Key |
JVMDQRDDGVKUMG-WOSURGFKSA-N | |
Source | PubChem | |
URL | https://pubchem.ncbi.nlm.nih.gov | |
Description | Data deposited in or computed by PubChem | |
Canonical SMILES |
C1=CC=C(C=C1)CC(C(=O)NC(CC2=CC=CC=C2)C(=O)NC(CCCN=C(N)N)C(=O)CCl)N.C(=O)(C(F)(F)F)O.C(=O)(C(F)(F)F)O | |
Source | PubChem | |
URL | https://pubchem.ncbi.nlm.nih.gov | |
Description | Data deposited in or computed by PubChem | |
Isomeric SMILES |
C1=CC=C(C=C1)C[C@H](C(=O)N[C@@H](CC2=CC=CC=C2)C(=O)N[C@@H](CCCN=C(N)N)C(=O)CCl)N.C(=O)(C(F)(F)F)O.C(=O)(C(F)(F)F)O | |
Source | PubChem | |
URL | https://pubchem.ncbi.nlm.nih.gov | |
Description | Data deposited in or computed by PubChem | |
Molecular Formula |
C29H35ClF6N6O7 | |
Source | PubChem | |
URL | https://pubchem.ncbi.nlm.nih.gov | |
Description | Data deposited in or computed by PubChem | |
Molecular Weight |
729.1 g/mol | |
Source | PubChem | |
URL | https://pubchem.ncbi.nlm.nih.gov | |
Description | Data deposited in or computed by PubChem | |
Retrosynthesis Analysis
AI-Powered Synthesis Planning: Our tool employs the Template_relevance Pistachio, Template_relevance Bkms_metabolic, Template_relevance Pistachio_ringbreaker, Template_relevance Reaxys, Template_relevance Reaxys_biocatalysis model, leveraging a vast database of chemical reactions to predict feasible synthetic routes.
One-Step Synthesis Focus: Specifically designed for one-step synthesis, it provides concise and direct routes for your target compounds, streamlining the synthesis process.
Accurate Predictions: Utilizing the extensive PISTACHIO, BKMS_METABOLIC, PISTACHIO_RINGBREAKER, REAXYS, REAXYS_BIOCATALYSIS database, our tool offers high-accuracy predictions, reflecting the latest in chemical research and data.
Strategy Settings
Precursor scoring | Relevance Heuristic |
---|---|
Min. plausibility | 0.01 |
Model | Template_relevance |
Template Set | Pistachio/Bkms_metabolic/Pistachio_ringbreaker/Reaxys/Reaxys_biocatalysis |
Top-N result to add to graph | 6 |
Feasible Synthetic Routes
Disclaimer and Information on In-Vitro Research Products
Please be aware that all articles and product information presented on BenchChem are intended solely for informational purposes. The products available for purchase on BenchChem are specifically designed for in-vitro studies, which are conducted outside of living organisms. In-vitro studies, derived from the Latin term "in glass," involve experiments performed in controlled laboratory settings using cells or tissues. It is important to note that these products are not categorized as medicines or drugs, and they have not received approval from the FDA for the prevention, treatment, or cure of any medical condition, ailment, or disease. We must emphasize that any form of bodily introduction of these products into humans or animals is strictly prohibited by law. It is essential to adhere to these guidelines to ensure compliance with legal and ethical standards in research and experimentation.