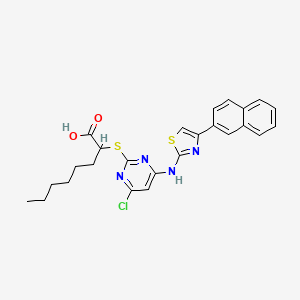
Pirinixic Acid Aminothiazole
Overview
Description
Pirinixic acid aminothiazole is a compound that combines the structural features of pirinixic acid and aminothiazole. Pirinixic acid is known for its role as a peroxisome proliferator-activated receptor alpha agonist, while aminothiazole is a versatile scaffold in medicinal chemistry with various biological activities. The combination of these two moieties results in a compound with potential therapeutic applications, particularly in the fields of inflammation and cancer .
Mechanism of Action
Target of Action
Pirinixic Acid Aminothiazole primarily targets two critical enzymes involved in the metabolism of arachidonic acid: 5-lipoxygenase (5-LO) and microsomal prostaglandin E2 synthase-1 (mPGES-1) . These enzymes play key roles in the synthesis of leukotrienes (LTs) and prostaglandin E2 (PGE2), respectively .
Mode of Action
This compound acts as a dual inhibitor of 5-LO and mPGES-1 . It interacts with these targets, suppressing their activity and thereby reducing the production of LTs and PGE2 . Importantly, it has been found to have significantly less pronounced inhibition of cyclooxygenases-1/2 (COX-1/2) activities .
Biochemical Pathways
The compound’s action affects the prostaglandin (PG) and leukotriene (LT) biosynthetic pathways . By inhibiting 5-LO and mPGES-1, it reduces the synthesis of LTs and PGE2, bioactive lipids that contribute to a broad range of pathologies, including inflammation and various forms of cancer .
Result of Action
The molecular and cellular effects of this compound’s action include reduced vascular permeability and inflammatory cell infiltration . In a zymosan-induced mouse peritonitis model, it was found to significantly impair levels of cysteinyl-leukotrienes and prostaglandin E2 .
Biochemical Analysis
Biochemical Properties
Pirinixic Acid Aminothiazole interacts with key enzymes, proteins, and other biomolecules in biochemical reactions. It is a potent inhibitor of both 5-LO and mPGES-1, with IC50 values of 0.3 and 0.4 μM, respectively . These enzymes are key players in the synthesis of leukotrienes (LTs) and PGE2, respectively . The nature of these interactions involves the compound binding to the active sites of these enzymes, thereby inhibiting their activity.
Cellular Effects
The effects of this compound on cells are significant. In cell-based assays, it directly inhibits 5-LO with an IC50 value of 0.2 μM . This inhibition can lead to a reduction in the synthesis of LTs and PGE2, which are involved in various cellular processes, including cell signaling pathways, gene expression, and cellular metabolism .
Molecular Mechanism
The molecular mechanism of action of this compound involves its binding interactions with biomolecules, enzyme inhibition, and changes in gene expression. As a dual inhibitor of 5-LO and mPGES-1, it binds to the active sites of these enzymes, inhibiting their activity and subsequently reducing the synthesis of LTs and PGE2 . This can lead to changes in gene expression and cellular function.
Temporal Effects in Laboratory Settings
Its potent inhibitory effects on 5-LO and mPGES-1 suggest that it may have long-term effects on cellular function, particularly in the context of inflammation and cancer .
Metabolic Pathways
This compound is involved in the arachidonic acid metabolic pathway, where it inhibits the enzymes 5-LO and mPGES-1 . These enzymes are key players in the synthesis of LTs and PGE2, respectively .
Preparation Methods
Synthetic Routes and Reaction Conditions: The synthesis of pirinixic acid aminothiazole typically involves the condensation of a halogenated ketone with a thioamide, followed by cyclization to form the aminothiazole ring. The reaction conditions often require the use of a base, such as sodium hydroxide, and a solvent like ethanol. The process can be optimized by using polymer-supported catalysts to improve yields and simplify product isolation .
Industrial Production Methods: Industrial production of this compound may involve large-scale batch reactions using similar synthetic routes. The use of continuous flow reactors and solid-supported catalysts can enhance efficiency and scalability. The final product is typically purified through crystallization or chromatography to achieve high purity .
Chemical Reactions Analysis
Types of Reactions: Pirinixic acid aminothiazole undergoes various chemical reactions, including:
Oxidation: The compound can be oxidized using reagents like hydrogen peroxide or potassium permanganate.
Reduction: Reduction reactions can be carried out using agents such as sodium borohydride.
Substitution: Nucleophilic substitution reactions can occur at the halogenated positions of the aminothiazole ring
Common Reagents and Conditions:
Oxidation: Hydrogen peroxide in acetic acid.
Reduction: Sodium borohydride in methanol.
Substitution: Sodium hydroxide in ethanol
Major Products: The major products formed from these reactions depend on the specific conditions and reagents used. For example, oxidation can yield sulfoxides or sulfones, while reduction can produce amines .
Scientific Research Applications
Pirinixic acid aminothiazole has a wide range of scientific research applications:
Chemistry: Used as a building block for the synthesis of more complex molecules.
Biology: Studied for its role in modulating enzyme activity and receptor binding.
Medicine: Investigated for its anti-inflammatory and anticancer properties. .
Industry: Utilized in the development of new pharmaceuticals and agrochemicals
Comparison with Similar Compounds
Pirinixic acid aminothiazole can be compared with other similar compounds, such as:
Fenofibrate: Another PPARα agonist used to lower lipid levels.
Clofibric Acid: A PPARα agonist with similar lipid-lowering effects but lacks the dual inhibitory action on mPGES-1 and 5-LO.
Thiazolidinediones: A class of PPARγ agonists used in diabetes treatment.
The uniqueness of this compound lies in its dual inhibitory action on mPGES-1 and 5-LO, combined with its PPARα agonist activity, making it a promising candidate for treating inflammatory diseases and cancer .
Properties
IUPAC Name |
2-[4-chloro-6-[(4-naphthalen-2-yl-1,3-thiazol-2-yl)amino]pyrimidin-2-yl]sulfanyloctanoic acid | |
---|---|---|
Source | PubChem | |
URL | https://pubchem.ncbi.nlm.nih.gov | |
Description | Data deposited in or computed by PubChem | |
InChI |
InChI=1S/C25H25ClN4O2S2/c1-2-3-4-5-10-20(23(31)32)34-25-28-21(26)14-22(30-25)29-24-27-19(15-33-24)18-12-11-16-8-6-7-9-17(16)13-18/h6-9,11-15,20H,2-5,10H2,1H3,(H,31,32)(H,27,28,29,30) | |
Source | PubChem | |
URL | https://pubchem.ncbi.nlm.nih.gov | |
Description | Data deposited in or computed by PubChem | |
InChI Key |
BADFIDKPYNKNRT-UHFFFAOYSA-N | |
Source | PubChem | |
URL | https://pubchem.ncbi.nlm.nih.gov | |
Description | Data deposited in or computed by PubChem | |
Canonical SMILES |
CCCCCCC(C(=O)O)SC1=NC(=CC(=N1)Cl)NC2=NC(=CS2)C3=CC4=CC=CC=C4C=C3 | |
Source | PubChem | |
URL | https://pubchem.ncbi.nlm.nih.gov | |
Description | Data deposited in or computed by PubChem | |
Molecular Formula |
C25H25ClN4O2S2 | |
Source | PubChem | |
URL | https://pubchem.ncbi.nlm.nih.gov | |
Description | Data deposited in or computed by PubChem | |
Molecular Weight |
513.1 g/mol | |
Source | PubChem | |
URL | https://pubchem.ncbi.nlm.nih.gov | |
Description | Data deposited in or computed by PubChem | |
Retrosynthesis Analysis
AI-Powered Synthesis Planning: Our tool employs the Template_relevance Pistachio, Template_relevance Bkms_metabolic, Template_relevance Pistachio_ringbreaker, Template_relevance Reaxys, Template_relevance Reaxys_biocatalysis model, leveraging a vast database of chemical reactions to predict feasible synthetic routes.
One-Step Synthesis Focus: Specifically designed for one-step synthesis, it provides concise and direct routes for your target compounds, streamlining the synthesis process.
Accurate Predictions: Utilizing the extensive PISTACHIO, BKMS_METABOLIC, PISTACHIO_RINGBREAKER, REAXYS, REAXYS_BIOCATALYSIS database, our tool offers high-accuracy predictions, reflecting the latest in chemical research and data.
Strategy Settings
Precursor scoring | Relevance Heuristic |
---|---|
Min. plausibility | 0.01 |
Model | Template_relevance |
Template Set | Pistachio/Bkms_metabolic/Pistachio_ringbreaker/Reaxys/Reaxys_biocatalysis |
Top-N result to add to graph | 6 |
Feasible Synthetic Routes
Disclaimer and Information on In-Vitro Research Products
Please be aware that all articles and product information presented on BenchChem are intended solely for informational purposes. The products available for purchase on BenchChem are specifically designed for in-vitro studies, which are conducted outside of living organisms. In-vitro studies, derived from the Latin term "in glass," involve experiments performed in controlled laboratory settings using cells or tissues. It is important to note that these products are not categorized as medicines or drugs, and they have not received approval from the FDA for the prevention, treatment, or cure of any medical condition, ailment, or disease. We must emphasize that any form of bodily introduction of these products into humans or animals is strictly prohibited by law. It is essential to adhere to these guidelines to ensure compliance with legal and ethical standards in research and experimentation.