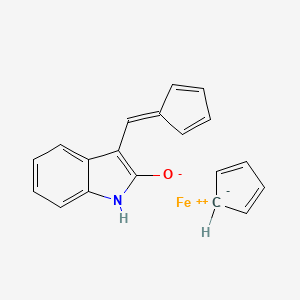
(Z)-FeCP-oxindole
Overview
Description
Selective inhibitor of human vascular endothelial cell growth factor receptor 2 (VEGFR-2) (IC50 = 220 nM). Displays anticancer activity (IC50< 1 μM against B16 murine melanoma lines). Does not significantly inhibit VEGFR1 or PDGFRa or b at a concentration of 10μM.
Scientific Research Applications
Synthesis and Electrochemical Studies
- Synthesis and Anticancer Activity : A series of (Z)-ferrocenyl oxindoles demonstrated promising anticancer activity, particularly against human breast cancer cells. The most potent Z isomers displayed significant potential for cancer therapy development (Silva et al., 2010).
Environmental and Remediation Studies
- Remediation Systems Efficiency : The efficiency of Fe0 for water remediation was significantly affected by the presence, amount, and age of iron corrosion products (FeCPs), impacting the removal efficiency of contaminants in Fe0/H2O systems (Touomo-Wouafo et al., 2018).
- Electrochemical Monitoring in Fe0/H2O Systems : The removal process of metal ions using Fe0 was monitored electrochemically. The study provided insights into the mutual interference of cations and the role of FeCPs in the removal process (Touomo-Wouafo et al., 2020).
Material Science and Chemistry
- Molecule-Based Magnets : Research on hybrid organometallic-inorganic layered magnets, including those formed by bimetallic oxalate-based layers separated by layers of organometallic cations, showed novel examples of magnetic materials with specific ordering temperatures (Coronado et al., 2001).
- Mn(V)-oxo Complex Synthesis : A high-spin Mn(V)-oxo complex was prepared from an oxomanganese(III) complex, providing insights into biological and synthetic processes involving oxomanganese species (Taguchi et al., 2012).
- Iron-Catalyzed Oxidative Carboacylation : A new oxidative tandem route to assemble 3-(2-oxoethyl)indolin-2-ones from N-arylacrylamides and alcohols using iron catalysts was established, important for oxindole synthesis (Ouyang et al., 2014).
- Cyclization of o-alkynylaryl Isocyanates : Research on the cyclization of o-(arylethynyl)aryl isocyanates led to the formation of 3-(arylchloromethylene)oxindoles, useful for further chemical transformations (Cantagrel et al., 2009).
- Bioorganometallic Kinase Inhibitors : A series of oxindole-containing ferrocenes were synthesized and tested for kinase inhibition, showing activities against specific isoforms and providing insights for drug discovery (Amin et al., 2013).
Medical and Pharmaceutical Research
- Biological Activities of Oxindole Derivatives : Oxindole derivatives exhibit a range of pharmacological implications, including anticancer, antimicrobial, and antiviral activities. The versatility of oxindole in medicinal chemistry was highlighted in this comprehensive review (Khetmalis et al., 2021).
Other Applications
- Heterobimetallic Complexes with Cu-Fe and Zn-Fe Bonds : The study synthesized heterobimetallic complexes, providing insights into metal-metal cooperativity and applications in catalysis (Jayarathne et al., 2013).
- Sonodynamic-Chemo Effects on Tumors : A metal-organic nanosonosensitizer was developed for noninvasive treatment of deep-seated tumors, showcasing the potential of FeCPs in medical applications (Xu et al., 2020).
Properties
IUPAC Name |
cyclopenta-1,3-diene;3-(cyclopenta-2,4-dien-1-ylidenemethyl)-1H-indol-2-olate;iron(2+) | |
---|---|---|
Source | PubChem | |
URL | https://pubchem.ncbi.nlm.nih.gov | |
Description | Data deposited in or computed by PubChem | |
InChI |
InChI=1S/C14H11NO.C5H5.Fe/c16-14-12(9-10-5-1-2-6-10)11-7-3-4-8-13(11)15-14;1-2-4-5-3-1;/h1-9,15-16H;1-5H;/q;-1;+2/p-1 | |
Source | PubChem | |
URL | https://pubchem.ncbi.nlm.nih.gov | |
Description | Data deposited in or computed by PubChem | |
InChI Key |
MTGBTDOTYGXLBK-UHFFFAOYSA-M | |
Source | PubChem | |
URL | https://pubchem.ncbi.nlm.nih.gov | |
Description | Data deposited in or computed by PubChem | |
Canonical SMILES |
[CH-]1C=CC=C1.C1=CC=C2C(=C1)C(=C(N2)[O-])C=C3C=CC=C3.[Fe+2] | |
Source | PubChem | |
URL | https://pubchem.ncbi.nlm.nih.gov | |
Description | Data deposited in or computed by PubChem | |
Molecular Formula |
C19H15FeNO | |
Source | PubChem | |
URL | https://pubchem.ncbi.nlm.nih.gov | |
Description | Data deposited in or computed by PubChem | |
Molecular Weight |
329.2 g/mol | |
Source | PubChem | |
URL | https://pubchem.ncbi.nlm.nih.gov | |
Description | Data deposited in or computed by PubChem | |
Retrosynthesis Analysis
AI-Powered Synthesis Planning: Our tool employs the Template_relevance Pistachio, Template_relevance Bkms_metabolic, Template_relevance Pistachio_ringbreaker, Template_relevance Reaxys, Template_relevance Reaxys_biocatalysis model, leveraging a vast database of chemical reactions to predict feasible synthetic routes.
One-Step Synthesis Focus: Specifically designed for one-step synthesis, it provides concise and direct routes for your target compounds, streamlining the synthesis process.
Accurate Predictions: Utilizing the extensive PISTACHIO, BKMS_METABOLIC, PISTACHIO_RINGBREAKER, REAXYS, REAXYS_BIOCATALYSIS database, our tool offers high-accuracy predictions, reflecting the latest in chemical research and data.
Strategy Settings
Precursor scoring | Relevance Heuristic |
---|---|
Min. plausibility | 0.01 |
Model | Template_relevance |
Template Set | Pistachio/Bkms_metabolic/Pistachio_ringbreaker/Reaxys/Reaxys_biocatalysis |
Top-N result to add to graph | 6 |
Feasible Synthetic Routes
Disclaimer and Information on In-Vitro Research Products
Please be aware that all articles and product information presented on BenchChem are intended solely for informational purposes. The products available for purchase on BenchChem are specifically designed for in-vitro studies, which are conducted outside of living organisms. In-vitro studies, derived from the Latin term "in glass," involve experiments performed in controlled laboratory settings using cells or tissues. It is important to note that these products are not categorized as medicines or drugs, and they have not received approval from the FDA for the prevention, treatment, or cure of any medical condition, ailment, or disease. We must emphasize that any form of bodily introduction of these products into humans or animals is strictly prohibited by law. It is essential to adhere to these guidelines to ensure compliance with legal and ethical standards in research and experimentation.