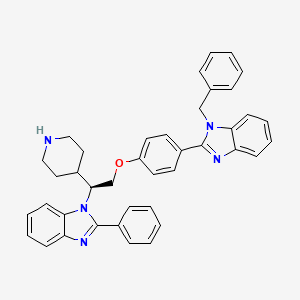
Deltarasin
- Click on QUICK INQUIRY to receive a quote from our team of experts.
- With the quality product at a COMPETITIVE price, you can focus more on your research.
Overview
Description
Deltarasin is a small molecule inhibitor that targets the interaction between the KRAS protein and phosphodiesterase delta (PDEδ). This compound has garnered significant attention due to its potential therapeutic applications in cancer treatment, particularly in cancers driven by KRAS mutations .
Mechanism of Action
Target of Action
Deltarasin primarily targets the KRAS-PDEδ interaction . KRAS is a protein that regulates cell growth and division, and its mutations are often associated with various types of cancer . PDEδ is a protein that binds to and actively shuttles KRAS to the correct location in plasma membranes, where it can then activate various signaling pathways required for the initiation and maintenance of cancer .
Mode of Action
This compound inhibits the KRAS-PDEδ interactions by binding to a hydrophobic pocket on PDEδ . This binding results in the disruption of KRAS signaling . The disruption of the KRAS–PDEδ interaction abrogates oncogenic signaling by KRAS .
Biochemical Pathways
The disruption of KRAS localization by this compound affects its signaling capacity. It reduces the levels of the downstream kinases extracellular signal-regulated kinase 1 (ERK1) and ERK2 . This compound induces apoptosis by inhibiting the interaction of KRAS with PDEδ and its downstream signaling pathways, while it induces autophagy through the AMPK-mTOR signaling pathway .
Pharmacokinetics
It’s known that this compound has nanomolar potency as well as sufficient solubility and membrane permeability . Further clinical studies are required to test its toxicity and efficiency in patients .
Result of Action
This compound produces both apoptosis and autophagy in KRAS-dependent lung cancer cells in vitro and inhibits lung tumor growth in vivo . It induces apoptosis via elevation of reactive oxygen species (ROS). In contrast, inhibition of ROS significantly attenuates this compound-induced cell death .
Action Environment
The action of this compound can be enhanced by simultaneously blocking tumor protective autophagy, but inhibited if combined with an antioxidant The environment, therefore, plays a crucial role in the efficacy of this compound
Biochemical Analysis
Biochemical Properties
Deltarasin binds to the farnesyl-binding pocket of PDEδ in cells, inhibiting its interaction with KRAS and disrupting RAS signaling . This interaction is crucial for the plasma membrane localization of prenylated Ras .
Cellular Effects
This compound has been reported to prevent KRAS plasma membrane localization and subsequent RAS/RAF signaling in human pancreatic ductal adenocarcinoma cell lines . It also produces apoptosis and autophagy in KRAS-dependent lung cancer cells in vitro .
Molecular Mechanism
This compound exerts its effects at the molecular level by inhibiting the interaction of PDEδ with KRAS and its downstream signaling pathways . It also induces autophagy through the AMPK-mTOR signaling pathway .
Temporal Effects in Laboratory Settings
Over time, this compound has been observed to inhibit lung tumor growth in vivo
Metabolic Pathways
It is known that this compound affects the AMPK-mTOR signaling pathway, which plays a key role in cellular metabolism .
Transport and Distribution
This compound is known to affect the localization of KRAS, preventing its accumulation at the plasma membrane
Subcellular Localization
This compound affects the subcellular localization of KRAS, preventing its enrichment at the plasma membrane
Preparation Methods
Synthetic Routes and Reaction Conditions
Deltarasin is synthesized through a multi-step organic synthesis process. The key steps involve the formation of the core structure, followed by functional group modifications to enhance its binding affinity and specificity for PDEδ. The synthetic route typically includes:
Formation of the Core Structure: This involves the construction of the central heterocyclic ring system.
Functional Group Modifications: Introduction of various substituents to improve the compound’s pharmacokinetic properties and binding affinity.
Industrial Production Methods
While specific industrial production methods for this compound are not widely documented, the general approach would involve scaling up the laboratory synthesis process. This includes optimizing reaction conditions, such as temperature, pressure, and solvent choice, to ensure high yield and purity of the final product .
Chemical Reactions Analysis
Types of Reactions
Deltarasin undergoes several types of chemical reactions, including:
Substitution Reactions: These reactions involve the replacement of one functional group with another, often to enhance the compound’s activity or stability.
Oxidation and Reduction Reactions: These reactions are used to modify the oxidation state of the compound, which can affect its biological activity.
Common Reagents and Conditions
Common reagents used in the synthesis and modification of this compound include:
Oxidizing Agents: Such as hydrogen peroxide or potassium permanganate.
Reducing Agents: Such as sodium borohydride or lithium aluminum hydride.
Major Products Formed
The major products formed from these reactions are various derivatives of this compound, each with potentially different biological activities and pharmacokinetic properties .
Scientific Research Applications
Deltarasin has a wide range of scientific research applications, including:
Cancer Research: this compound is primarily used in cancer research to study its effects on KRAS-driven cancers. .
Biological Studies: Researchers use this compound to investigate the role of KRAS in cellular signaling pathways and its impact on cell proliferation and apoptosis.
Drug Development: This compound serves as a lead compound for the development of new cancer therapeutics targeting the KRAS-PDEδ interaction.
Comparison with Similar Compounds
Similar Compounds
Farnesylthiosalicylic Acid (FTS): Another inhibitor of the RAS protein, but with a different mechanism of action.
Kobe0065: A small molecule that also targets the RAS protein but through a different binding site.
Uniqueness of Deltarasin
This compound is unique in its high specificity and affinity for the PDEδ binding pocket, making it a potent inhibitor of the KRAS-PDEδ interaction. This specificity reduces off-target effects and enhances its potential as a therapeutic agent .
Properties
IUPAC Name |
1-benzyl-2-[4-[(2S)-2-(2-phenylbenzimidazol-1-yl)-2-piperidin-4-ylethoxy]phenyl]benzimidazole |
Source
|
---|---|---|
Source | PubChem | |
URL | https://pubchem.ncbi.nlm.nih.gov | |
Description | Data deposited in or computed by PubChem | |
InChI |
InChI=1S/C40H37N5O/c1-3-11-29(12-4-1)27-44-36-17-9-7-15-34(36)42-39(44)32-19-21-33(22-20-32)46-28-38(30-23-25-41-26-24-30)45-37-18-10-8-16-35(37)43-40(45)31-13-5-2-6-14-31/h1-22,30,38,41H,23-28H2/t38-/m1/s1 |
Source
|
Source | PubChem | |
URL | https://pubchem.ncbi.nlm.nih.gov | |
Description | Data deposited in or computed by PubChem | |
InChI Key |
LTZKEDSUXKTTTC-KXQOOQHDSA-N |
Source
|
Source | PubChem | |
URL | https://pubchem.ncbi.nlm.nih.gov | |
Description | Data deposited in or computed by PubChem | |
Canonical SMILES |
C1CNCCC1C(COC2=CC=C(C=C2)C3=NC4=CC=CC=C4N3CC5=CC=CC=C5)N6C7=CC=CC=C7N=C6C8=CC=CC=C8 |
Source
|
Source | PubChem | |
URL | https://pubchem.ncbi.nlm.nih.gov | |
Description | Data deposited in or computed by PubChem | |
Isomeric SMILES |
C1CNCCC1[C@@H](COC2=CC=C(C=C2)C3=NC4=CC=CC=C4N3CC5=CC=CC=C5)N6C7=CC=CC=C7N=C6C8=CC=CC=C8 |
Source
|
Source | PubChem | |
URL | https://pubchem.ncbi.nlm.nih.gov | |
Description | Data deposited in or computed by PubChem | |
Molecular Formula |
C40H37N5O |
Source
|
Source | PubChem | |
URL | https://pubchem.ncbi.nlm.nih.gov | |
Description | Data deposited in or computed by PubChem | |
Molecular Weight |
603.8 g/mol |
Source
|
Source | PubChem | |
URL | https://pubchem.ncbi.nlm.nih.gov | |
Description | Data deposited in or computed by PubChem | |
Disclaimer and Information on In-Vitro Research Products
Please be aware that all articles and product information presented on BenchChem are intended solely for informational purposes. The products available for purchase on BenchChem are specifically designed for in-vitro studies, which are conducted outside of living organisms. In-vitro studies, derived from the Latin term "in glass," involve experiments performed in controlled laboratory settings using cells or tissues. It is important to note that these products are not categorized as medicines or drugs, and they have not received approval from the FDA for the prevention, treatment, or cure of any medical condition, ailment, or disease. We must emphasize that any form of bodily introduction of these products into humans or animals is strictly prohibited by law. It is essential to adhere to these guidelines to ensure compliance with legal and ethical standards in research and experimentation.