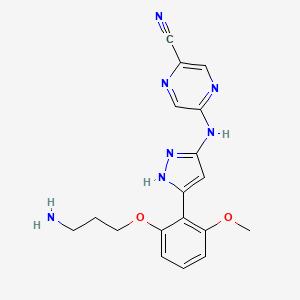
Prexasertib
Overview
Description
Prexasertib is a small molecule checkpoint kinase inhibitor, primarily targeting checkpoint kinase 1 (CHEK1) with minor activity against checkpoint kinase 2 (CHEK2). This compound induces DNA double-strand breaks, leading to apoptosis (programmed cell death). It was developed by Eli Lilly and has shown potential in treating various cancers, including acute myeloid leukemia, myelodysplastic syndrome, rhabdomyosarcoma, and medulloblastoma .
Mechanism of Action
- This disruption leads to the induction of DNA double-strand breaks, ultimately resulting in apoptosis .
- Prexasertib affects several pathways:
- Early clinical studies show efficacy when combined with other drugs, such as antimetabolites, PARP inhibitors, and platinum-based chemotherapy .
Target of Action
Mode of Action
Biochemical Pathways
Result of Action
Action Environment
Biochemical Analysis
Biochemical Properties
Prexasertib interacts with the multifunctional proteins of the kinase family, CHK1 and CHK2 . These proteins play a crucial role in regulating DNA replication and DNA damage response . When a cell is exposed to exogenous damage to its DNA, CHK1/CHK2 stops the cell cycle to give time to the cellular mechanisms to repair DNA breakage .
Cellular Effects
This compound has shown to induce DNA damage and apoptosis in tumor cells . It influences cell function by impacting cell signaling pathways, gene expression, and cellular metabolism .
Molecular Mechanism
This compound exerts its effects at the molecular level by inhibiting CHK1 and CHK2 . This inhibition leads to the induction of DNA double-strand breaks, resulting in apoptosis .
Temporal Effects in Laboratory Settings
In preclinical studies, this compound has shown to induce DNA damage and tumor cells apoptosis .
Dosage Effects in Animal Models
The effects of this compound vary with different dosages in animal models
Metabolic Pathways
This compound is involved in the DNA damage response pathways . It interacts with the ataxia telangiectasia and Rad3-related kinase (ATR) or ataxia telangiectasia mutated kinase (ATM) in response to DNA replication stress or DNA damage .
Preparation Methods
Prexasertib is synthesized through a series of chemical reactions involving pyrazole and pyrazine derivativesThe reaction conditions often include the use of organic solvents, catalysts, and controlled temperatures to ensure optimal yield and purity .
Industrial production methods for this compound involve continuous-flow solid-phase synthesis, which allows for efficient and scalable production. This method improves mass and heat transfer, enhances mixing efficiency, and reduces the risk of side-product formation .
Chemical Reactions Analysis
Prexasertib undergoes various chemical reactions, including:
Oxidation: this compound can be oxidized to form different derivatives, which may exhibit varying biological activities.
Reduction: Reduction reactions can modify the functional groups on this compound, potentially altering its pharmacological properties.
Substitution: Substitution reactions involve replacing specific atoms or groups within the this compound molecule with other functional groups, leading to the formation of new derivatives.
Common reagents and conditions used in these reactions include organic solvents, catalysts, and controlled temperatures. The major products formed from these reactions are typically derivatives of this compound with modified functional groups .
Scientific Research Applications
Chemistry: Prexasertib serves as a valuable tool for studying the mechanisms of DNA damage and repair, as well as the role of checkpoint kinases in cell cycle regulation.
Biology: Researchers use this compound to investigate the cellular responses to DNA damage and the pathways involved in apoptosis.
Medicine: this compound has shown promise in treating various cancers, including acute myeloid leukemia, myelodysplastic syndrome, rhabdomyosarcoma, and medulloblastoma.
Industry: The compound’s ability to induce DNA damage and apoptosis makes it a potential candidate for developing new cancer therapies and improving existing treatment regimens
Comparison with Similar Compounds
Prexasertib belongs to a class of compounds known as checkpoint kinase inhibitors. Similar compounds include:
Rabusertib: Another CHEK1 inhibitor with potential anticancer activity.
SRA737: A selective CHEK1 inhibitor that has shown promise in preclinical and clinical studies.
LY2606368: A CHEK1/2 inhibitor with similar mechanisms of action to this compound.
This compound is unique in its ability to induce DNA double-strand breaks and apoptosis, making it a valuable tool for studying DNA damage and repair mechanisms.
Properties
IUPAC Name |
5-[[5-[2-(3-aminopropoxy)-6-methoxyphenyl]-1H-pyrazol-3-yl]amino]pyrazine-2-carbonitrile | |
---|---|---|
Source | PubChem | |
URL | https://pubchem.ncbi.nlm.nih.gov | |
Description | Data deposited in or computed by PubChem | |
InChI |
InChI=1S/C18H19N7O2/c1-26-14-4-2-5-15(27-7-3-6-19)18(14)13-8-16(25-24-13)23-17-11-21-12(9-20)10-22-17/h2,4-5,8,10-11H,3,6-7,19H2,1H3,(H2,22,23,24,25) | |
Source | PubChem | |
URL | https://pubchem.ncbi.nlm.nih.gov | |
Description | Data deposited in or computed by PubChem | |
InChI Key |
DOTGPNHGTYJDEP-UHFFFAOYSA-N | |
Source | PubChem | |
URL | https://pubchem.ncbi.nlm.nih.gov | |
Description | Data deposited in or computed by PubChem | |
Canonical SMILES |
COC1=C(C(=CC=C1)OCCCN)C2=CC(=NN2)NC3=NC=C(N=C3)C#N | |
Source | PubChem | |
URL | https://pubchem.ncbi.nlm.nih.gov | |
Description | Data deposited in or computed by PubChem | |
Molecular Formula |
C18H19N7O2 | |
Source | PubChem | |
URL | https://pubchem.ncbi.nlm.nih.gov | |
Description | Data deposited in or computed by PubChem | |
DSSTOX Substance ID |
DTXSID301031326 | |
Record name | Prexasertib | |
Source | EPA DSSTox | |
URL | https://comptox.epa.gov/dashboard/DTXSID301031326 | |
Description | DSSTox provides a high quality public chemistry resource for supporting improved predictive toxicology. | |
Molecular Weight |
365.4 g/mol | |
Source | PubChem | |
URL | https://pubchem.ncbi.nlm.nih.gov | |
Description | Data deposited in or computed by PubChem | |
CAS No. |
1234015-52-1 | |
Record name | 5-[[5-[2-(3-Aminopropoxy)-6-methoxyphenyl]-1H-pyrazol-3-yl]amino]-2-pyrazinecarbonitrile | |
Source | CAS Common Chemistry | |
URL | https://commonchemistry.cas.org/detail?cas_rn=1234015-52-1 | |
Description | CAS Common Chemistry is an open community resource for accessing chemical information. Nearly 500,000 chemical substances from CAS REGISTRY cover areas of community interest, including common and frequently regulated chemicals, and those relevant to high school and undergraduate chemistry classes. This chemical information, curated by our expert scientists, is provided in alignment with our mission as a division of the American Chemical Society. | |
Explanation | The data from CAS Common Chemistry is provided under a CC-BY-NC 4.0 license, unless otherwise stated. | |
Record name | Prexasertib [USAN] | |
Source | ChemIDplus | |
URL | https://pubchem.ncbi.nlm.nih.gov/substance/?source=chemidplus&sourceid=1234015521 | |
Description | ChemIDplus is a free, web search system that provides access to the structure and nomenclature authority files used for the identification of chemical substances cited in National Library of Medicine (NLM) databases, including the TOXNET system. | |
Record name | Prexasertib | |
Source | DrugBank | |
URL | https://www.drugbank.ca/drugs/DB12008 | |
Description | The DrugBank database is a unique bioinformatics and cheminformatics resource that combines detailed drug (i.e. chemical, pharmacological and pharmaceutical) data with comprehensive drug target (i.e. sequence, structure, and pathway) information. | |
Explanation | Creative Common's Attribution-NonCommercial 4.0 International License (http://creativecommons.org/licenses/by-nc/4.0/legalcode) | |
Record name | Prexasertib | |
Source | EPA DSSTox | |
URL | https://comptox.epa.gov/dashboard/DTXSID301031326 | |
Description | DSSTox provides a high quality public chemistry resource for supporting improved predictive toxicology. | |
Record name | Prexasertib | |
Source | FDA Global Substance Registration System (GSRS) | |
URL | https://gsrs.ncats.nih.gov/ginas/app/beta/substances/820NH671E6 | |
Description | The FDA Global Substance Registration System (GSRS) enables the efficient and accurate exchange of information on what substances are in regulated products. Instead of relying on names, which vary across regulatory domains, countries, and regions, the GSRS knowledge base makes it possible for substances to be defined by standardized, scientific descriptions. | |
Explanation | Unless otherwise noted, the contents of the FDA website (www.fda.gov), both text and graphics, are not copyrighted. They are in the public domain and may be republished, reprinted and otherwise used freely by anyone without the need to obtain permission from FDA. Credit to the U.S. Food and Drug Administration as the source is appreciated but not required. | |
Q1: What is Prexasertib and what is its primary mechanism of action?
A1: this compound (LY2606368) is a potent, selective, small-molecule inhibitor of checkpoint kinase proteins 1 (CHK1) and 2 (CHK2). [] CHK1 and CHK2 are serine/threonine kinases that play critical roles in the DNA damage response (DDR) pathway and cell cycle regulation. [] By inhibiting these kinases, this compound disrupts cell cycle checkpoints, particularly the G2/M and S-phase checkpoints, leading to increased replication stress, DNA damage accumulation, and ultimately, cell death. [, , ]
Q2: How does this compound's inhibition of CHK1 and CHK2 lead to tumor cell death?
A2: this compound's inhibition of CHK1 and CHK2 prevents the proper activation of cell cycle checkpoints, which are crucial for DNA repair and cell cycle arrest in response to DNA damage. This disruption leads to several downstream effects that contribute to tumor cell death:
- Increased Replication Stress: Cells with impaired checkpoint function are unable to properly manage replication stress, a state of stalled or collapsed DNA replication forks. This results in the accumulation of DNA damage and ultimately, cell death. [, , ]
- Mitotic Catastrophe: Inhibition of CHK1/2 forces cells with DNA damage to progress through the cell cycle prematurely, leading to mitotic catastrophe. This is a type of cell death characterized by aberrant mitotic events and the formation of multinucleated, non-viable cells. [, ]
- Impaired DNA Repair: this compound has been shown to hinder homologous recombination (HR), a key DNA repair pathway. [, , ] By blocking HR, this compound further sensitizes cancer cells to DNA damage and cell death.
Q3: What are the potential advantages of targeting CHK1/2 in cancer therapy?
A3: Targeting CHK1/2 presents several potential advantages in cancer therapy:
- Synthetic Lethality: Many cancer cells have inherent defects in DNA repair pathways, making them more reliant on CHK1/2-mediated checkpoint activation for survival. [, , ] Inhibiting CHK1/2 in these cells can exploit this vulnerability and induce selective cancer cell death.
- Chemosensitization: Preclinical studies have demonstrated that this compound can enhance the efficacy of conventional chemotherapy and other targeted therapies by abrogating DNA damage checkpoints and increasing the vulnerability of cancer cells to DNA-damaging agents. [, , , , ]
- Tumor Microenvironment Modulation: this compound has been shown to influence the tumor immune microenvironment by increasing the expression of genes associated with T-cell activation and immune cell trafficking while decreasing the expression of immunosuppressive genes. [] This suggests a potential for combining this compound with immunotherapies.
Q4: Is there any information available about this compound's molecular formula, weight, or spectroscopic data?
A4: Detailed structural information about this compound, including its molecular formula, weight, and spectroscopic data, is not provided in the provided abstracts. Further investigation into patent literature or chemical databases may be necessary to obtain this information.
Q5: Are there any details available regarding the material compatibility, stability under various conditions, catalytic properties, computational chemistry studies, or structure-activity relationship (SAR) of this compound?
A5: The provided abstracts primarily focus on this compound's preclinical and clinical development as a CHK1/2 inhibitor in various cancer models. They do not provide details on material compatibility, stability under various conditions, catalytic properties, computational chemistry studies, or SAR. Further research may be required to gather such information.
Q6: What is known about this compound's pharmacokinetic profile?
A6: Several studies provide insights into this compound's PK profile:
- Absorption & Distribution: Following intravenous administration in humans, this compound exhibits dose-independent and time-independent pharmacokinetics. []
- Metabolism: this compound undergoes extensive metabolism, primarily via oxidative deamination, O-dealkylation, mono-oxidation, and potentially direct glucuronide conjugation. [] Unchanged this compound represents a minor fraction of total radioactivity in plasma, indicating the presence of circulating metabolites. []
- Excretion: Both renal and fecal excretion contribute significantly to this compound elimination. Approximately 42% of the administered dose is recovered in urine and 32% in feces. []
- Half-Life: In humans, the geometric mean plasma half-life of this compound is 34.2 hours, while total radioactivity exhibits a half-life of 73.8 hours. []
Q7: What preclinical models have been used to assess this compound's anticancer activity?
A7: this compound's anticancer activity has been extensively evaluated in diverse preclinical models, including:
- Cell Lines: Numerous cancer cell lines, representing various tumor types like triple-negative breast cancer (TNBC) [, , ], neuroblastoma [, ], osteosarcoma [, ], Ewing sarcoma [], rhabdomyosarcoma [], small cell lung cancer (SCLC) [, ], head and neck squamous cell carcinoma (HNSCC) [, ], pancreatic cancer [, ], and others, have been utilized to assess this compound's in vitro efficacy.
- Xenograft Models: Both cell line-derived xenografts (CDX) and patient-derived xenografts (PDX) have been employed to evaluate this compound's in vivo activity in various cancers, including TNBC [, , ], neuroblastoma [], rhabdomyosarcoma [], osteosarcoma [], Ewing sarcoma [], and others.
- Orthotopic Models: Orthotopic models, where tumor cells are implanted into their corresponding organ of origin, have also been used to assess this compound's efficacy in mimicking the tumor microenvironment more closely. [, ]
Q8: What are the key findings from preclinical studies of this compound?
A8: Preclinical studies have demonstrated the following key findings regarding this compound:
- Single-Agent Activity: this compound exhibits promising single-agent activity in various preclinical models, particularly in tumors with inherent defects in DNA repair pathways or those exhibiting high replication stress. [, , ]
- Synergistic Combinations: Combining this compound with conventional chemotherapies (e.g., gemcitabine, cisplatin), PARP inhibitors (e.g., olaparib, talazoparib), or other targeted therapies (e.g., PI3K/mTOR inhibitors) often results in synergistic or additive antitumor effects in preclinical models. [, , , , , , , , ]
- Overcoming Resistance: Preclinical data suggest that combining this compound with other agents may help overcome or prevent the emergence of resistance to single-agent therapies. [, ]
Q9: Have there been any clinical trials involving this compound, and what are the key findings?
A9: Yes, several clinical trials have investigated this compound as a potential cancer treatment:
- Phase I Trials: Phase I trials in adults with advanced solid tumors have established the maximum tolerated dose (MTD) and safety profile of this compound. [, ] These trials have also provided evidence of single-agent activity in various cancers, including head and neck squamous cell carcinoma (HNSCC) [], squamous cell carcinoma of the anus (SCCA) [], and others.
- Phase II Trials: A phase II trial in patients with BRCA wild-type, platinum-resistant, recurrent high-grade serous ovarian cancer (HGSOC) demonstrated promising activity of this compound monotherapy. [] Additionally, a phase II study in BRCA wild-type, advanced triple-negative breast cancer (TNBC) showed modest activity of this compound as a single agent. []
- Combination Trials: Several clinical trials are ongoing or have been completed evaluating this compound in combination with other agents, such as PARP inhibitors (e.g., olaparib) and PI3K/mTOR inhibitors (e.g., samotolisib), based on promising preclinical findings. [, , ]
Q10: What are the known mechanisms of resistance to this compound?
A10: While this compound shows promise in preclinical and clinical settings, acquired resistance remains a challenge. Studies have identified several potential resistance mechanisms:
- WEE1 Upregulation: Increased expression of WEE1, another cell cycle regulator, has been linked to acquired resistance to this compound in small cell lung cancer (SCLC). [] This suggests that combining CHK1 and WEE1 inhibitors could potentially overcome this resistance mechanism.
- EGFR Signaling: Activation of the epidermal growth factor receptor (EGFR) pathway has been implicated in innate resistance to this compound in triple-negative breast cancer (TNBC). [] EGFR activation promotes the phosphorylation of BAD, inactivating its pro-apoptotic functions and contributing to resistance.
- Innate Immunity Genes: Transcriptome analysis has linked the expression of certain innate immunity genes to this compound resistance. [] This suggests a potential role for the tumor microenvironment and immune evasion in mediating resistance to CHK1 inhibition.
Q11: What is the safety profile of this compound based on preclinical and clinical studies?
A11: this compound has generally been well-tolerated in clinical trials, with manageable side effects.
- Hematologic Toxicity: The most common adverse events observed in clinical trials are hematologic toxicities, primarily neutropenia, anemia, and thrombocytopenia. [, , ] These side effects are usually manageable with supportive care measures, such as granulocyte colony-stimulating factor (G-CSF) support.
- Other Adverse Events: Other less common side effects reported in clinical trials include nausea, fatigue, vomiting, stomatitis, and febrile neutropenia. [, , ]
Q12: What is known about drug delivery strategies, target specificity, biomarkers for efficacy prediction, and diagnostic applications related to this compound?
A12: The provided abstracts primarily focus on this compound's preclinical and clinical activity as a CHK1/2 inhibitor. While they do not provide in-depth information on drug delivery strategies, target specificity beyond CHK1/2 inhibition, or diagnostic applications, they highlight several areas of interest:
- Biomarkers of Response: Identifying reliable biomarkers to predict response to this compound is crucial for patient selection and optimizing treatment outcomes. Preclinical studies have explored potential biomarkers, including:
- Replication Stress: Tumors exhibiting high levels of replication stress may be more sensitive to CHK1/2 inhibition. []
- DNA Repair Deficiencies: Defects in specific DNA repair pathways, such as homologous recombination (HR), may enhance sensitivity to this compound. []
- Cyclin E Dysregulation: While cyclin E dysregulation has been implicated in this compound response, its predictive power as a biomarker requires further investigation. []
- Pharmacodynamic (PD) Markers: Clinical trials have explored PD markers to monitor this compound's activity, including:
- DNA Damage: Increased levels of DNA damage markers, such as γH2AX, pKAP1, and pRPA, have been observed in tumor biopsies following this compound treatment. []
- Immune Modulation: Changes in immune cell subsets, such as an increase in monocytic myeloid-derived suppressor cells (M-MDSCs), have been associated with this compound treatment. []
Disclaimer and Information on In-Vitro Research Products
Please be aware that all articles and product information presented on BenchChem are intended solely for informational purposes. The products available for purchase on BenchChem are specifically designed for in-vitro studies, which are conducted outside of living organisms. In-vitro studies, derived from the Latin term "in glass," involve experiments performed in controlled laboratory settings using cells or tissues. It is important to note that these products are not categorized as medicines or drugs, and they have not received approval from the FDA for the prevention, treatment, or cure of any medical condition, ailment, or disease. We must emphasize that any form of bodily introduction of these products into humans or animals is strictly prohibited by law. It is essential to adhere to these guidelines to ensure compliance with legal and ethical standards in research and experimentation.