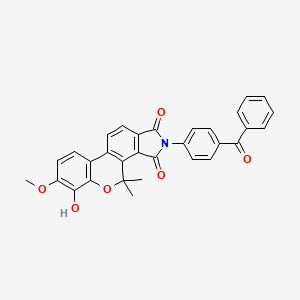
Ampkinone
- Click on QUICK INQUIRY to receive a quote from our team of experts.
- With the quality product at a COMPETITIVE price, you can focus more on your research.
Overview
Description
Ampkinone is an AMP-activated protein kinase (AMPK) activator . AMPK plays a role in energy metabolism and glucose homeostasis by monitoring the ratio of ATP to AMP .
Synthesis Analysis
The formal name of Ampkinone is 2-(4-benzoylphenyl)-6-hydroxy-7-methoxy-4,4-dimethyl-1benzopyrano[3,4-e]isoindole-1,3 (2H,4H)-dione . It has a molecular formula of C31H23NO6 .
Molecular Structure Analysis
Ampkinone has a molecular weight of 505.52 and a molecular formula of C31H23NO6 . The structure of Ampkinone is complex and involves various functional groups .
Physical And Chemical Properties Analysis
Ampkinone is a solid compound with a light yellow to yellow color . It has a molecular weight of 505.52 and a molecular formula of C31H23NO6 .
Scientific Research Applications
Treatment of Metabolic Disorders
Ampkinone is a novel small molecule that indirectly activates AMP-activated protein kinase (AMPK), which has emerged as an attractive target molecule for the treatment of metabolic disorders, including obesity and type 2 diabetes .
Antiobesity Effects
Ampkinone has shown potential antiobesity effects. In a study, ampkinone-treated DIO mice significantly reduced total body weight and overall fat mass .
Antidiabetic Effects
Ampkinone has potential antidiabetic effects. It stimulates the phosphorylation of AMPK, resulting in increased glucose uptake in muscle cells .
Improvement of Metabolic Abnormalities
Ampkinone effectively improved metabolic abnormalities in the DIO mice model. This suggests its potential use in the treatment of various metabolic disorders .
Activation of AMPK
Ampkinone is a small molecule activator of AMPK. AMPK stimulates glucose uptake in skeletal muscle when activated during muscle contraction and exercise .
Potential Therapeutic Agent for Diabetes and Obesity
Ampkinone, a small molecule with a privileged benzopyran substructure, has potential as a new class of therapeutic agent for antidiabetic and antiobesity treatment via the indirect stimulation of AMPK .
Flavonoid Activation of AMPK
Ampkinone is one of the flavonoids that show strong AMPK activation. This suggests its potential use in the development of drugs for diabetes and obesity .
Potential Role in Exercise and Muscle Contraction
AMPK, which Ampkinone activates, is stimulated during muscle contraction and exercise, suggesting a potential role of Ampkinone in these processes .
Mechanism of Action
Target of Action
Ampkinone primarily targets the AMP-activated protein kinase (AMPK) . AMPK is a central regulator of energy homeostasis, coordinating metabolic pathways to balance nutrient supply with energy demand . It is a serine/threonine protein kinase complex consisting of a catalytic α-subunit, a scaffolding β-subunit, and a regulatory γ-subunit .
Safety and Hazards
properties
IUPAC Name |
2-(4-benzoylphenyl)-6-hydroxy-7-methoxy-4,4-dimethylchromeno[3,4-e]isoindole-1,3-dione |
Source
|
---|---|---|
Source | PubChem | |
URL | https://pubchem.ncbi.nlm.nih.gov | |
Description | Data deposited in or computed by PubChem | |
InChI |
InChI=1S/C31H23NO6/c1-31(2)25-20(21-15-16-23(37-3)27(34)28(21)38-31)13-14-22-24(25)30(36)32(29(22)35)19-11-9-18(10-12-19)26(33)17-7-5-4-6-8-17/h4-16,34H,1-3H3 |
Source
|
Source | PubChem | |
URL | https://pubchem.ncbi.nlm.nih.gov | |
Description | Data deposited in or computed by PubChem | |
InChI Key |
BRRCHDIWBKOMEC-UHFFFAOYSA-N |
Source
|
Source | PubChem | |
URL | https://pubchem.ncbi.nlm.nih.gov | |
Description | Data deposited in or computed by PubChem | |
Canonical SMILES |
CC1(C2=C(C=CC3=C2C(=O)N(C3=O)C4=CC=C(C=C4)C(=O)C5=CC=CC=C5)C6=C(O1)C(=C(C=C6)OC)O)C |
Source
|
Source | PubChem | |
URL | https://pubchem.ncbi.nlm.nih.gov | |
Description | Data deposited in or computed by PubChem | |
Molecular Formula |
C31H23NO6 |
Source
|
Source | PubChem | |
URL | https://pubchem.ncbi.nlm.nih.gov | |
Description | Data deposited in or computed by PubChem | |
Molecular Weight |
505.5 g/mol |
Source
|
Source | PubChem | |
URL | https://pubchem.ncbi.nlm.nih.gov | |
Description | Data deposited in or computed by PubChem | |
Disclaimer and Information on In-Vitro Research Products
Please be aware that all articles and product information presented on BenchChem are intended solely for informational purposes. The products available for purchase on BenchChem are specifically designed for in-vitro studies, which are conducted outside of living organisms. In-vitro studies, derived from the Latin term "in glass," involve experiments performed in controlled laboratory settings using cells or tissues. It is important to note that these products are not categorized as medicines or drugs, and they have not received approval from the FDA for the prevention, treatment, or cure of any medical condition, ailment, or disease. We must emphasize that any form of bodily introduction of these products into humans or animals is strictly prohibited by law. It is essential to adhere to these guidelines to ensure compliance with legal and ethical standards in research and experimentation.