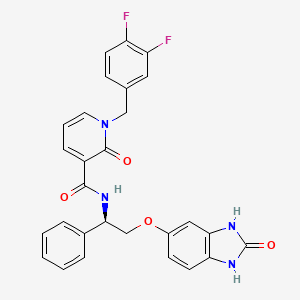
PDK1 inhibitor
Overview
Description
Pyruvate dehydrogenase kinase 1 inhibitors are compounds that inhibit the activity of pyruvate dehydrogenase kinase 1, an enzyme that plays a crucial role in cellular metabolism. Pyruvate dehydrogenase kinase 1 is responsible for phosphorylating and inactivating pyruvate dehydrogenase, which in turn regulates the conversion of pyruvate to acetyl coenzyme A, a key step in the tricarboxylic acid cycle. By inhibiting pyruvate dehydrogenase kinase 1, these compounds can modulate cellular energy metabolism, making them valuable in the treatment of various diseases, including cancer, diabetes, and cardiovascular diseases .
Preparation Methods
Synthetic Routes and Reaction Conditions: The synthesis of pyruvate dehydrogenase kinase 1 inhibitors often involves the creation of small molecule libraries through various synthetic routes. One common approach is the design and synthesis of 3-amino-1,2,4-triazine derivatives. These compounds are synthesized using a molecular hybridization approach, which involves the combination of different chemical moieties to create a library of potential inhibitors . The reaction conditions typically involve the use of organic solvents, catalysts, and specific temperature and pressure conditions to optimize the yield and purity of the final product.
Industrial Production Methods: Industrial production of pyruvate dehydrogenase kinase 1 inhibitors involves scaling up the synthetic routes used in laboratory settings. This often requires the optimization of reaction conditions to ensure consistent quality and yield. Techniques such as high-performance liquid chromatography and mass spectrometry are used to monitor the purity and concentration of the inhibitors. Additionally, the use of automated synthesis platforms can enhance the efficiency and scalability of the production process .
Chemical Reactions Analysis
Pyrrolo-Pyridine Derivatives via Click Chemistry
-
Reaction : Copper(I)-catalyzed azide-alkyne cycloaddition ([3+2] cycloaddition) forms triazole-linked pyrrolo-pyridine derivatives.
-
Example : Inhibitors such as compound XXX (Table 1) exhibit PDK1 IC<sub>50</sub> values of 0.5 nM–1 μM in vitro, with cell-based IC<sub>50</sub> values of 1–10 μM .
-
Selectivity : These compounds also inhibit IKKε but show minimal cross-reactivity with unrelated kinases .
Aminoindazole-Based Inhibitors via Suzuki Coupling
-
Reaction : Suzuki-Miyaura coupling of 4-chloropyrimidine intermediates with boronic esters, followed by hydrazine-mediated cyclization .
-
Example : GSK2334470 (Table 1) binds the PDK1 hinge region via hydrogen bonds with Ser160 and Ala162, achieving nanomolar potency .
-
Optimization : Structural modifications at the pyrimidine 6-position enhance hydrophobic interactions with the PDK1 G-loop .
ATP-Competitive vs. Allosteric Inhibition
-
Allosteric Activation : PDK1-tide1 fusion peptides enhance substrate affinity by 50-fold compared to AKT(Thr-308)-tide, enabling high-throughput screening .
-
Cooperative Binding : PIP<sub>3</sub> induces PDK1 dimerization, facilitating trans-autophosphorylation at Ser241 .
Kinetic Parameters of PDK1 Substrates
-
Substrate Affinity : K<sub>m</sub> = 40 nM for PDK1-tide1 fusion peptide .
-
Mechanism : Rapid equilibrium random bireactant reaction, confirmed via biophysical assays .
Thermodynamic Binding Studies
-
2-O-Bn-InsP<sub>5</sub> : Binds PDK1 PH domain with K<sub>d</sub> = 109 nM (ΔH = −4.1 kcal/mol), showing no affinity for AKT2 PH domain .
-
Crystallographic Data : Compound 7 occupies the PDK1 DFG-out pocket, disrupting T-loop phosphorylation (Ser241) .
Functional Consequences of Inhibition
-
Cellular Effects : Selective PDK1 inhibitors (e.g., compound 7 ) impair anchorage-independent growth and metastasis in 40–60% of cancer lines .
-
Biomarkers : Phospho-PDK1 Ser241 and phospho-AKT Thr308 serve as pharmacodynamic markers .
-
Synergy : MAPK4 enhances PDK1 protein synthesis, suggesting combined targeting strategies in triple-negative breast cancer .
Scientific Research Applications
Colorectal Cancer
A study demonstrated that silencing PDK1 expression significantly reduced liver metastasis in colorectal cancer models. The combination of PDK1 knockdown with cryptotanshinone, an inhibitor of STAT3 phosphorylation, further inhibited metastasis and increased apoptosis in colorectal cancer cells . This suggests that targeting PDK1 could enhance therapeutic efficacy against metastatic colorectal cancer.
Multiple Myeloma
Dichloroacetate (DCA), a known PDK1 inhibitor, has been shown to induce apoptosis in multiple myeloma cells without affecting normal peripheral blood mononuclear cells (PBMCs). In vitro studies indicated that DCA treatment led to increased reactive oxygen species (ROS) production and activation of caspase-3, which are markers of apoptosis . The findings highlight the potential of DCA as a selective therapeutic agent for multiple myeloma patients.
Other Cancer Types
PDK1 inhibitors have been investigated across various cancer types, including gliomas and breast cancer. Clinical trials are ongoing to evaluate the safety and efficacy of these inhibitors in combination with other therapies . The selective targeting of PDK1 may provide a strategic advantage over broader inhibitors that affect multiple pathways.
Table 1: Summary of Case Studies on PDK1 Inhibitors
Challenges and Future Directions
Despite the promising results from preclinical studies, several challenges remain in the clinical application of PDK1 inhibitors:
- Selectivity : Many available inhibitors lack specificity, leading to off-target effects. The development of highly selective inhibitors is crucial for minimizing side effects while maximizing therapeutic benefits .
- Resistance Mechanisms : Tumor cells may develop resistance to PDK1 inhibition through alternative survival pathways. Combination therapies that target multiple pathways may be necessary to overcome this challenge .
- Biomarker Development : Identifying biomarkers predictive of response to PDK1 inhibitors will be essential for patient stratification in clinical trials .
Mechanism of Action
The mechanism of action of pyruvate dehydrogenase kinase 1 inhibitors involves the inhibition of pyruvate dehydrogenase kinase 1 activity, which prevents the phosphorylation and inactivation of pyruvate dehydrogenase. This leads to an increase in the conversion of pyruvate to acetyl coenzyme A, enhancing the tricarboxylic acid cycle and oxidative phosphorylation . The molecular targets of these inhibitors include the active site of pyruvate dehydrogenase kinase 1 and other regulatory proteins involved in cellular metabolism .
Comparison with Similar Compounds
Pyruvate dehydrogenase kinase 1 inhibitors can be compared with other similar compounds, such as inhibitors of other pyruvate dehydrogenase kinase isoforms (e.g., pyruvate dehydrogenase kinase 2, pyruvate dehydrogenase kinase 3, and pyruvate dehydrogenase kinase 4). While all these inhibitors target the pyruvate dehydrogenase kinase family, pyruvate dehydrogenase kinase 1 inhibitors are unique in their ability to specifically modulate the activity of pyruvate dehydrogenase kinase 1, leading to distinct effects on cellular metabolism .
Similar compounds include dichloroacetic acid, which inhibits multiple pyruvate dehydrogenase kinase isoforms, and other small molecule inhibitors that target specific isoforms of pyruvate dehydrogenase kinase . The uniqueness of pyruvate dehydrogenase kinase 1 inhibitors lies in their selectivity and potency, making them valuable tools for studying and modulating cellular metabolism .
Biological Activity
Phosphoinositide-dependent kinase 1 (PDK1) is a pivotal regulator in various cellular processes, particularly in cancer biology. As a member of the AGC kinase family, PDK1 activates several downstream kinases, including AKT, which are crucial for cell survival, proliferation, and metabolism. The development of selective PDK1 inhibitors has opened new avenues for therapeutic interventions in cancer and other diseases. This article explores the biological activity of PDK1 inhibitors, highlighting their mechanisms, efficacy in preclinical and clinical studies, and potential applications.
PDK1 functions by phosphorylating a conserved threonine residue in the activation loop of AGC kinases, which is essential for their activation. This phosphorylation event is critical for the regulation of several signaling pathways involved in oncogenesis and cell survival. The inhibition of PDK1 disrupts these pathways, leading to decreased activity of downstream targets such as AKT, which can induce apoptosis and inhibit tumor growth.
Selective PDK1 Inhibitors
Recent research has identified various selective PDK1 inhibitors that exhibit distinct binding properties and mechanisms:
- Compound 7 : This inhibitor uniquely binds to the inactive conformation of PDK1 (DFG-out), selectively inhibiting T-loop phosphorylation at Ser-241. It has shown promise in impairing anchorage-independent growth and migration in cancer cell lines .
- Dichloroacetate (DCA) : Initially studied for its metabolic effects, DCA has been reported to induce apoptosis in multiple myeloma (MM) cells through PDK1 inhibition. It demonstrated dose-dependent cytotoxicity while sparing normal cells .
Efficacy in Cancer Models
Several studies have demonstrated the efficacy of PDK1 inhibitors across various cancer types:
- Multiple Myeloma : Inhibition of PDK1 using DCA led to significant apoptosis in MM cell lines and primary cells, with minimal toxicity to normal peripheral blood mononuclear cells (PBMCs). This indicates a therapeutic window for targeting PDK1 in hematological malignancies .
- Breast Cancer : Co-targeting PDK1 and AKT has been shown to effectively repress tumor growth driven by MAPK4 signaling in triple-negative breast cancer (TNBC) models. This suggests that dual inhibition may enhance therapeutic outcomes .
Case Studies
The following table summarizes key findings from research studies on the biological activity of PDK1 inhibitors:
Pharmacological Insights
The pharmacological characterization of PDK1 inhibitors reveals their potential as targeted therapies. Genetic studies have shown that loss of PDK1 results in decreased kinase activity across multiple pathways, underscoring its role as a "master regulator" within the AGC family . Furthermore, profiling studies indicate that selective inhibition can lead to significant effects on tumorigenesis without broadly affecting normal cellular functions.
Q & A
Basic Research Questions
Q. What experimental strategies are recommended to validate PDK1 inhibitor specificity in cellular models?
Methodological Answer:
- Perform kinase profiling assays to assess off-target effects. For example, BX-795 inhibits PDK1 (IC₅₀ = ~10 nM) but also targets TBK1 and IKKε, requiring validation via siRNA knockdown or rescue experiments with constitutively active PDK1 .
- Use phospho-specific antibodies (e.g., p-Akt Thr308) to confirm downstream pathway inhibition. GSK2334470 reduces Akt phosphorylation by >80% at 2 μM in melanoma models .
- Combine with PDK1-deficient cell lines (e.g., PDK1 hypomorphic mice-derived cells) to confirm on-target effects .
Q. How do PDK1 inhibitors modulate the PI3K/Akt/mTOR pathway in cancer research?
Methodological Answer:
- PDK1 inhibitors block Akt activation by preventing phosphorylation at Thr307. For example, MP7 (HY-14440) reduces Akt activity by 70% in NSCLC cells, measured via immunoblotting .
- Assess cross-talk with MAPK pathways : Dual MEK/PDK1 inhibitors (e.g., compound 9za) synergize with PD0325901 (MEK inhibitor) to induce apoptosis in lung cancer models .
- Monitor metabolic effects via Seahorse assays , as PDK1 inhibition alters glycolysis (e.g., dichloroacetate (DCA) reduces lactate production by 50% in Taxol-resistant oral cancer cells) .
Q. What are the standard assays for evaluating this compound efficacy in vitro?
Methodological Answer:
- Kinase activity assays : Use recombinant PDK1 and Akt substrates (IC₅₀ values for GSK2334470 = 10–30 nM) .
- Anchorage-independent growth assays : BX-795 reduces colony formation by 60–80% in breast cancer cell lines .
- Apoptosis assays : OSU-03012 increases caspase-3/7 activity by 3-fold in glioblastoma models .
Advanced Research Questions
Q. How can researchers address contradictory data on this compound efficacy across cancer types?
Methodological Answer:
- Analyze tissue-specific PDK1 expression (e.g., PDK1 is overexpressed in melanoma but not pancreatic cancer) .
- Investigate compensatory pathways : MAPK4 activation rescues PDK1/Akt inhibition in prostate cancer, requiring co-targeting with MK2206 (Akt inhibitor) .
- Use patient-derived xenografts (PDX) to model heterogeneity. For example, MP7 shows variable IC₅₀ values (0.2–5 μM) across 60 cancer cell lines .
Q. What experimental designs are optimal for studying this compound resistance mechanisms?
Methodological Answer:
- Generate long-term resistant clones via gradual dose escalation (e.g., Taxol-resistant oral cancer cells upregulate PDK1 mRNA by 4-fold) .
- Perform RNA-seq to identify upregulated bypass pathways (e.g., mTORC1 activation in PDK1-inhibited cells) .
- Test combination therapies : Co-treatment with DCA and Taxol reduces tumor volume by 90% in resistant xenografts .
Q. How should researchers validate this compound binding modes and structural interactions?
Methodological Answer:
- Conduct molecular docking studies using PDK1’s kinase domain (PDB ID: 1W1G). Virtual screening identified a novel inhibitor (IC₅₀ = 200 nM) with >90% binding similarity to ATP .
- Use surface plasmon resonance (SPR) to measure binding kinetics. BAG-956 shows KD = 8 nM for PDK1 .
- Validate via mutagenesis : Replace Ser241 in PDK1’s activation loop to disrupt inhibitor binding .
Q. What in vivo models are suitable for assessing this compound toxicity and efficacy?
Methodological Answer:
- PDK1 hypomorphic mice : Exhibit 80–90% PDK1 reduction without lethal cardiac phenotypes, ideal for toxicity studies .
- Metastasis models : this compound GSK2334470 delays melanoma metastasis by >50% in syngeneic mouse models .
- Monitor glucose metabolism : DCA-treated mice show reduced blood lactate levels (1.5 mM vs. 3.2 mM in controls) .
Properties
IUPAC Name |
1-[(3,4-difluorophenyl)methyl]-2-oxo-N-[(1R)-2-[(2-oxo-1,3-dihydrobenzimidazol-5-yl)oxy]-1-phenylethyl]pyridine-3-carboxamide | |
---|---|---|
Source | PubChem | |
URL | https://pubchem.ncbi.nlm.nih.gov | |
Description | Data deposited in or computed by PubChem | |
InChI |
InChI=1S/C28H22F2N4O4/c29-21-10-8-17(13-22(21)30)15-34-12-4-7-20(27(34)36)26(35)31-25(18-5-2-1-3-6-18)16-38-19-9-11-23-24(14-19)33-28(37)32-23/h1-14,25H,15-16H2,(H,31,35)(H2,32,33,37)/t25-/m0/s1 | |
Source | PubChem | |
URL | https://pubchem.ncbi.nlm.nih.gov | |
Description | Data deposited in or computed by PubChem | |
InChI Key |
GCWCGSPBENFEPE-VWLOTQADSA-N | |
Source | PubChem | |
URL | https://pubchem.ncbi.nlm.nih.gov | |
Description | Data deposited in or computed by PubChem | |
Canonical SMILES |
C1=CC=C(C=C1)C(COC2=CC3=C(C=C2)NC(=O)N3)NC(=O)C4=CC=CN(C4=O)CC5=CC(=C(C=C5)F)F | |
Source | PubChem | |
URL | https://pubchem.ncbi.nlm.nih.gov | |
Description | Data deposited in or computed by PubChem | |
Isomeric SMILES |
C1=CC=C(C=C1)[C@H](COC2=CC3=C(C=C2)NC(=O)N3)NC(=O)C4=CC=CN(C4=O)CC5=CC(=C(C=C5)F)F | |
Source | PubChem | |
URL | https://pubchem.ncbi.nlm.nih.gov | |
Description | Data deposited in or computed by PubChem | |
Molecular Formula |
C28H22F2N4O4 | |
Source | PubChem | |
URL | https://pubchem.ncbi.nlm.nih.gov | |
Description | Data deposited in or computed by PubChem | |
DSSTOX Substance ID |
DTXSID90678515 | |
Record name | 1-[(3,4-Difluorophenyl)methyl]-2-oxo-N-{(1R)-2-[(2-oxo-2,3-dihydro-1H-benzimidazol-5-yl)oxy]-1-phenylethyl}-1,2-dihydropyridine-3-carboxamide | |
Source | EPA DSSTox | |
URL | https://comptox.epa.gov/dashboard/DTXSID90678515 | |
Description | DSSTox provides a high quality public chemistry resource for supporting improved predictive toxicology. | |
Molecular Weight |
516.5 g/mol | |
Source | PubChem | |
URL | https://pubchem.ncbi.nlm.nih.gov | |
Description | Data deposited in or computed by PubChem | |
CAS No. |
1001409-50-2 | |
Record name | 1-[(3,4-Difluorophenyl)methyl]-2-oxo-N-{(1R)-2-[(2-oxo-2,3-dihydro-1H-benzimidazol-5-yl)oxy]-1-phenylethyl}-1,2-dihydropyridine-3-carboxamide | |
Source | EPA DSSTox | |
URL | https://comptox.epa.gov/dashboard/DTXSID90678515 | |
Description | DSSTox provides a high quality public chemistry resource for supporting improved predictive toxicology. | |
Disclaimer and Information on In-Vitro Research Products
Please be aware that all articles and product information presented on BenchChem are intended solely for informational purposes. The products available for purchase on BenchChem are specifically designed for in-vitro studies, which are conducted outside of living organisms. In-vitro studies, derived from the Latin term "in glass," involve experiments performed in controlled laboratory settings using cells or tissues. It is important to note that these products are not categorized as medicines or drugs, and they have not received approval from the FDA for the prevention, treatment, or cure of any medical condition, ailment, or disease. We must emphasize that any form of bodily introduction of these products into humans or animals is strictly prohibited by law. It is essential to adhere to these guidelines to ensure compliance with legal and ethical standards in research and experimentation.