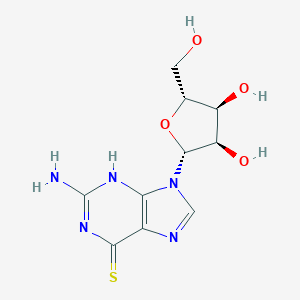
6-Thioguanosine
Overview
Description
Mechanism of Action
Target of Action
The primary target of 6-Thioguanosine is the enzyme hypoxanthine-guanine phosphoribosyltransferase (HGPRTase) . This enzyme plays a crucial role in the purine salvage pathway, which is essential for nucleotide synthesis .
Mode of Action
This compound interacts with HGPRTase and is converted into This compound monophosphate (TGMP) . TGMP is further metabolized into its diphosphate (TGDP) and triphosphate (TGTP) forms, collectively known as 6-thioguanine nucleotides (6-TGN) . These 6-TGNs exert cytotoxic effects on cells through two primary mechanisms:
- They incorporate into DNA during the synthesis phase (S-phase) of the cell cycle .
- They inhibit the GTP-binding protein (G protein) Rac1, which regulates the Rac/Vav pathway .
Biochemical Pathways
The biochemical pathways affected by this compound primarily involve DNA synthesis and the Rac/Vav pathway . The incorporation of 6-TGNs into DNA disrupts normal DNA replication and transcription, leading to DNA strand breaks . The inhibition of the Rac1 GTPase disrupts the regulation of the Rac/Vav pathway, which can lead to cytotoxic effects in cells .
Pharmacokinetics
The bioavailability of this compound is influenced by its metabolism by HGPRTase . After conversion to TGMP, it accumulates in cells and inhibits the conversion of inosinic acid to xanthylic acid
Result of Action
The molecular and cellular effects of this compound’s action include DNA damage, disruption of normal cell cycle progression, and cell death . The incorporation of 6-TGNs into DNA can trigger the post-replicative mismatch repair (MMR) pathway, leading to cell death . Additionally, the inhibition of the Rac1 GTPase can have cytotoxic effects on cells .
Action Environment
The action, efficacy, and stability of this compound can be influenced by various environmental factors. For instance, resistance to this compound can develop due to the expression of the salvage pathway enzyme HGPRT . Furthermore, the presence of this compound can diminish the thermodynamic stability of RNA duplexes, depending on its position within the duplexes and the sequence of adjacent base pairs .
Biochemical Analysis
Biochemical Properties
6-Thioguanosine interacts with the enzyme hypoxanthine-guanine phosphoribosyltransferase (HGPRTase) and gets converted into this compound monophosphate (TGMP) . The presence of this compound diminishes the thermodynamic stability of RNA duplexes . When placed at a dangling end, a this compound residue actually exerts a weak stabilizing effect .
Cellular Effects
This compound has been shown to have significant effects on various types of cells and cellular processes. It has been found to be effective against thiopurine-resistant leukemia and breast cancer cells . The cytotoxic effects of this compound are partly mediated by the metabolite this compound 5’-triphosphate, which inhibits the function of the small GTPase Rac1, leading to apoptosis of activated T cells .
Molecular Mechanism
This compound is a 6-thiopurine analogue of the naturally occurring purine bases hypoxanthine and guanine. Intracellular activation results in its incorporation into DNA as a false purine base . An additional cytotoxic effect is related to its incorporation into RNA . It competes with hypoxanthine and guanine for the enzyme HGPRTase and is itself converted to 6-thioguanilyic acid (TGMP), which reaches high intracellular concentrations at therapeutic doses .
Temporal Effects in Laboratory Settings
The presence of this compound causes only minor distortions in the overall structure of duplex DNA, these changes create the environment for cytotoxicity . The effects of this compound on purine biosynthesis and cell viability have been examined in H.Ep. 2 cells grown in culture .
Metabolic Pathways
This compound is converted by the enzyme HGPRTase to this compound monophosphate (TGMP). The biologically active compounds are deoxy-thioguanosine triphosphate (TdGTP) and thioguanosine triphosphate (TGTP) .
Subcellular Localization
The subcellular localization of this compound is not explicitly documented. Given its incorporation into DNA and RNA, it can be inferred that it localizes to the nucleus where these molecules reside .
Preparation Methods
Synthetic Routes and Reaction Conditions: Thioguanosine can be synthesized through various methods. One common approach involves the reaction of guanosine with thiophosphoryl chloride in the presence of a base, such as pyridine. The reaction typically occurs under mild conditions and results in the substitution of the oxygen atom at the 6-position of guanosine with a sulfur atom, forming thioguanosine .
Industrial Production Methods: Industrial production of thioguanosine often involves large-scale synthesis using similar chemical reactions. The process may include additional purification steps, such as recrystallization or chromatography, to ensure the high purity of the final product.
Chemical Reactions Analysis
Types of Reactions: Thioguanosine undergoes various chemical reactions, including oxidation, reduction, and substitution.
Common Reagents and Conditions:
Oxidation: Thioguanosine can be oxidized using reagents such as hydrogen peroxide or potassium permanganate.
Reduction: Reduction of thioguanosine can be achieved using reducing agents like sodium borohydride.
Substitution: Thioguanosine can undergo substitution reactions where the sulfur atom is replaced by other functional groups.
Major Products Formed: The major products formed from these reactions include sulfoxides, sulfones, and various substituted derivatives of thioguanosine.
Scientific Research Applications
Thioguanosine has a wide range of applications in scientific research:
Chemistry: It is used as a reagent in the synthesis of other sulfur-containing nucleosides and nucleotides.
Biology: Thioguanosine is employed in studies of nucleic acid interactions and modifications.
Comparison with Similar Compounds
Properties
IUPAC Name |
2-amino-9-[3,4-dihydroxy-5-(hydroxymethyl)oxolan-2-yl]-3H-purine-6-thione | |
---|---|---|
Details | Computed by Lexichem TK 2.7.0 (PubChem release 2021.05.07) | |
Source | PubChem | |
URL | https://pubchem.ncbi.nlm.nih.gov | |
Description | Data deposited in or computed by PubChem | |
InChI |
InChI=1S/C10H13N5O4S/c11-10-13-7-4(8(20)14-10)12-2-15(7)9-6(18)5(17)3(1-16)19-9/h2-3,5-6,9,16-18H,1H2,(H3,11,13,14,20) | |
Details | Computed by InChI 1.0.6 (PubChem release 2021.05.07) | |
Source | PubChem | |
URL | https://pubchem.ncbi.nlm.nih.gov | |
Description | Data deposited in or computed by PubChem | |
InChI Key |
OTDJAMXESTUWLO-UHFFFAOYSA-N | |
Details | Computed by InChI 1.0.6 (PubChem release 2021.05.07) | |
Source | PubChem | |
URL | https://pubchem.ncbi.nlm.nih.gov | |
Description | Data deposited in or computed by PubChem | |
Canonical SMILES |
C1=NC2=C(N1C3C(C(C(O3)CO)O)O)NC(=NC2=S)N | |
Details | Computed by OEChem 2.3.0 (PubChem release 2021.05.07) | |
Source | PubChem | |
URL | https://pubchem.ncbi.nlm.nih.gov | |
Description | Data deposited in or computed by PubChem | |
Molecular Formula |
C10H13N5O4S | |
Details | Computed by PubChem 2.1 (PubChem release 2021.05.07) | |
Source | PubChem | |
URL | https://pubchem.ncbi.nlm.nih.gov | |
Description | Data deposited in or computed by PubChem | |
DSSTOX Substance ID |
DTXSID20903307 | |
Record name | NoName_3952 | |
Source | EPA DSSTox | |
URL | https://comptox.epa.gov/dashboard/DTXSID20903307 | |
Description | DSSTox provides a high quality public chemistry resource for supporting improved predictive toxicology. | |
Molecular Weight |
299.31 g/mol | |
Details | Computed by PubChem 2.1 (PubChem release 2021.05.07) | |
Source | PubChem | |
URL | https://pubchem.ncbi.nlm.nih.gov | |
Description | Data deposited in or computed by PubChem | |
CAS No. |
32976-84-4, 26017-62-9, 32865-28-4, 7602-04-2, 85-31-4 | |
Record name | MLS000738229 | |
Source | DTP/NCI | |
URL | https://dtp.cancer.gov/dtpstandard/servlet/dwindex?searchtype=NSC&outputformat=html&searchlist=133418 | |
Description | The NCI Development Therapeutics Program (DTP) provides services and resources to the academic and private-sector research communities worldwide to facilitate the discovery and development of new cancer therapeutic agents. | |
Explanation | Unless otherwise indicated, all text within NCI products is free of copyright and may be reused without our permission. Credit the National Cancer Institute as the source. | |
Record name | 9H-Purine-6-thiol, monohydrate | |
Source | DTP/NCI | |
URL | https://dtp.cancer.gov/dtpstandard/servlet/dwindex?searchtype=NSC&outputformat=html&searchlist=123432 | |
Description | The NCI Development Therapeutics Program (DTP) provides services and resources to the academic and private-sector research communities worldwide to facilitate the discovery and development of new cancer therapeutic agents. | |
Explanation | Unless otherwise indicated, all text within NCI products is free of copyright and may be reused without our permission. Credit the National Cancer Institute as the source. | |
Record name | NSC109159 | |
Source | DTP/NCI | |
URL | https://dtp.cancer.gov/dtpstandard/servlet/dwindex?searchtype=NSC&outputformat=html&searchlist=109159 | |
Description | The NCI Development Therapeutics Program (DTP) provides services and resources to the academic and private-sector research communities worldwide to facilitate the discovery and development of new cancer therapeutic agents. | |
Explanation | Unless otherwise indicated, all text within NCI products is free of copyright and may be reused without our permission. Credit the National Cancer Institute as the source. | |
Record name | NSC89218 | |
Source | DTP/NCI | |
URL | https://dtp.cancer.gov/dtpstandard/servlet/dwindex?searchtype=NSC&outputformat=html&searchlist=89218 | |
Description | The NCI Development Therapeutics Program (DTP) provides services and resources to the academic and private-sector research communities worldwide to facilitate the discovery and development of new cancer therapeutic agents. | |
Explanation | Unless otherwise indicated, all text within NCI products is free of copyright and may be reused without our permission. Credit the National Cancer Institute as the source. | |
Record name | 6-Thioguanosine | |
Source | DTP/NCI | |
URL | https://dtp.cancer.gov/dtpstandard/servlet/dwindex?searchtype=NSC&outputformat=html&searchlist=29422 | |
Description | The NCI Development Therapeutics Program (DTP) provides services and resources to the academic and private-sector research communities worldwide to facilitate the discovery and development of new cancer therapeutic agents. | |
Explanation | Unless otherwise indicated, all text within NCI products is free of copyright and may be reused without our permission. Credit the National Cancer Institute as the source. | |
Retrosynthesis Analysis
AI-Powered Synthesis Planning: Our tool employs the Template_relevance Pistachio, Template_relevance Bkms_metabolic, Template_relevance Pistachio_ringbreaker, Template_relevance Reaxys, Template_relevance Reaxys_biocatalysis model, leveraging a vast database of chemical reactions to predict feasible synthetic routes.
One-Step Synthesis Focus: Specifically designed for one-step synthesis, it provides concise and direct routes for your target compounds, streamlining the synthesis process.
Accurate Predictions: Utilizing the extensive PISTACHIO, BKMS_METABOLIC, PISTACHIO_RINGBREAKER, REAXYS, REAXYS_BIOCATALYSIS database, our tool offers high-accuracy predictions, reflecting the latest in chemical research and data.
Strategy Settings
Precursor scoring | Relevance Heuristic |
---|---|
Min. plausibility | 0.01 |
Model | Template_relevance |
Template Set | Pistachio/Bkms_metabolic/Pistachio_ringbreaker/Reaxys/Reaxys_biocatalysis |
Top-N result to add to graph | 6 |
Feasible Synthetic Routes
Disclaimer and Information on In-Vitro Research Products
Please be aware that all articles and product information presented on BenchChem are intended solely for informational purposes. The products available for purchase on BenchChem are specifically designed for in-vitro studies, which are conducted outside of living organisms. In-vitro studies, derived from the Latin term "in glass," involve experiments performed in controlled laboratory settings using cells or tissues. It is important to note that these products are not categorized as medicines or drugs, and they have not received approval from the FDA for the prevention, treatment, or cure of any medical condition, ailment, or disease. We must emphasize that any form of bodily introduction of these products into humans or animals is strictly prohibited by law. It is essential to adhere to these guidelines to ensure compliance with legal and ethical standards in research and experimentation.