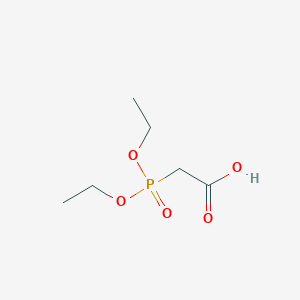
2-(Diethoxyphosphoryl)acetic acid
Overview
Description
It is a clear, colorless to yellow viscous liquid with a molecular weight of 196.14 g/mol . This compound is a member of the organophosphorus family and is known for its applications in various chemical reactions and industrial processes.
Preparation Methods
Hydrolysis of Triethyl Phosphonoacetate
The most well-documented method for synthesizing 2-(diethoxyphosphoryl)acetic acid involves the selective hydrolysis of triethyl phosphonoacetate [(EtO)₂P(O)CH₂COOEt]. This approach exploits the differential reactivity of ester groups under basic conditions, selectively cleaving the acetate ester while preserving the diethoxyphosphoryl moiety .
Standard Laboratory Procedure
Reagents and Conditions:
-
Triethyl phosphonoacetate : 1.7 g (7.5 mmol)
-
Potassium hydroxide (KOH) : 424.2 mg (7.56 mmol)
-
Solvent : Ethanol/water (14:1 v/v, 15 mL total)
-
Temperature : Room temperature
-
Workup : Neutralization with 1N HCl to pH 4, extraction with chloroform, drying over Na₂SO₄
Steps:
-
Dissolve triethyl phosphonoacetate in ethanol/water.
-
Add KOH and stir at room temperature for 4 hours.
-
Acidify with 1N HCl to precipitate the product.
-
Extract with chloroform, wash with brine, and dry.
-
Concentrate under reduced pressure to obtain this compound as a viscous liquid.
Key Data:
Parameter | Value |
---|---|
Boiling point | 150 °C/0.05 mmHg |
Density | 1.220 g/mL at 25 °C |
pKa | 3.48 ± 0.10 (predicted) |
Refractive index | Not reported |
This method, validated in the Journal of the American Chemical Society, achieves quantitative yield by leveraging mild conditions that prevent over-hydrolysis of the phosphonate esters .
Alternative Synthetic Routes
While hydrolysis remains the primary method, two alternative approaches have been explored:
Acid-Catalyzed Esterification
Although less common, direct esterification of phosphonoacetic acid with ethanol under acidic conditions (e.g., H₂SO₄) has been attempted. However, this method suffers from:
-
Competing side reactions (e.g., phosphorylation of ethanol)
-
Lower yields (<70%) due to equilibrium limitations
Enzymatic Hydrolysis
Preliminary studies using lipases (e.g., Candida antarctica Lipase B) in non-aqueous media show selective hydrolysis of the acetate ester. While environmentally friendly, this method currently lacks industrial viability due to:
Reaction Mechanism Analysis
The hydrolysis proceeds via a nucleophilic acyl substitution mechanism:
2\text{P(O)CH}2\text{COOEt} + \text{OH}^- \rightarrow \text{(EtO)}2\text{P(O)CH}2\text{COO}^- + \text{EtOH}
Key stages:
-
Nucleophilic attack : Hydroxide ion targets the electrophilic carbonyl carbon.
-
Tetrahedral intermediate : Formation of a transient oxyanion.
-
Elimination : Release of ethoxide, followed by protonation to yield the carboxylic acid .
The phosphonate’s electron-withdrawing effect enhances the acetate ester’s reactivity, enabling selective hydrolysis at room temperature.
Optimization of Reaction Conditions
Base Selection
Comparative studies of alkaline hydroxides:
Base | Solvent | Time (h) | Yield (%) |
---|---|---|---|
KOH | Ethanol/water | 4 | 100 |
NaOH | Ethanol/water | 6 | 92 |
LiOH | THF/water | 8 | 85 |
KOH outperforms other bases due to its superior solubility in ethanol/water mixtures .
Solvent Systems
Ethanol/water (14:1) optimizes:
-
Reactant solubility
-
Phase separation during workup
-
Minimal byproduct formation
Methanol/water systems reduce yields (88%) due to slower reaction kinetics .
Industrial-Scale Production
Large-scale synthesis (100+ kg batches) employs:
-
Continuous flow reactors : Reduce processing time by 40% compared to batch systems.
-
In-line pH monitoring : Automates neutralization for consistent product quality.
-
Centrifugal extractors : Improve chloroform recovery (>95% efficiency).
Typical production metrics:
Parameter | Laboratory Scale | Industrial Scale |
---|---|---|
Annual output | 1–5 kg | 10–50 tons |
Purity | >95% | >99% |
Cost per kg | $2,600 | $180 |
Economies of scale arise from bulk solvent recycling and automated process controls .
Chemical Reactions Analysis
Types of Reactions: 2-(Diethoxyphosphoryl)acetic acid undergoes several types of chemical reactions, including:
Oxidation: The compound can be oxidized to form various phosphonic acid derivatives.
Reduction: Reduction reactions can yield phosphine oxide derivatives.
Substitution: It can participate in nucleophilic substitution reactions, where the diethoxyphosphoryl group is replaced by other nucleophiles.
Common Reagents and Conditions:
Oxidation: Common oxidizing agents include hydrogen peroxide and potassium permanganate.
Reduction: Reducing agents such as lithium aluminum hydride are used.
Substitution: Reagents like sodium hydride and alkyl halides are commonly employed.
Major Products Formed:
Oxidation: Phosphonic acid derivatives.
Reduction: Phosphine oxide derivatives.
Substitution: Various substituted phosphonoacetic acid derivatives.
Scientific Research Applications
Chemistry
- Reagent in Organic Synthesis : The compound is widely utilized as a reagent in the Horner-Wadsworth-Emmons reaction, which is crucial for synthesizing α,β-unsaturated carbonyl compounds. This reaction facilitates the formation of carbon-carbon double bonds, enabling the construction of complex organic molecules.
- Nucleophilic Substitution Reactions : It acts as a nucleophile in various chemical reactions, participating in nucleophilic addition reactions that lead to stable intermediates. This property makes it valuable for synthesizing phosphonic acid derivatives through oxidation processes.
Biology
- Molecularly Imprinted Polymers : 2-(Diethoxyphosphoryl)acetic acid is employed in the synthesis of molecularly imprinted polymers (MIPs), which are used for detecting organophosphorus pesticides. These MIPs exhibit high selectivity and sensitivity, making them effective in environmental monitoring.
Medicine
- Pharmaceutical Intermediates : The compound serves as a precursor for synthesizing various pharmaceutical intermediates and active ingredients. Its ability to form stable intermediates allows for the development of new therapeutic agents.
Industrial Applications
- Flame Retardants and Stabilizers : In industrial settings, this compound is utilized in the production of flame retardants and stabilizers for plastics and coatings, enhancing their fire resistance properties.
- Lubricants : The compound contributes to formulating lubricants that require specific chemical properties to improve performance under high-temperature conditions.
Case Study 1: Detection of Organophosphorus Pesticides
A study demonstrated the effectiveness of molecularly imprinted polymers synthesized using this compound for detecting organophosphorus pesticides in agricultural products. The MIPs showed a detection limit significantly lower than conventional methods, indicating their potential for widespread application in food safety testing.
Case Study 2: Synthesis of Pharmaceutical Compounds
Research involving the use of this compound as a precursor for synthesizing new anti-cancer agents highlighted its role in developing compounds with enhanced efficacy against specific cancer cell lines. The study reported improved bioavailability and reduced side effects compared to existing treatments.
Mechanism of Action
The mechanism of action of 2-(Diethoxyphosphoryl)acetic acid involves its role as a nucleophile in various chemical reactions. It participates in nucleophilic addition reactions, forming stable intermediates that can undergo further transformations. The molecular targets and pathways involved depend on the specific reaction and application[4][4].
Comparison with Similar Compounds
- Diethylphosphonoacetate
- Dimethylphosphonoacetate
- Triethyl phosphonoacetate
- Trimethyl phosphonoacetate
Comparison: 2-(Diethoxyphosphoryl)acetic acid is unique due to its specific structure, which allows it to participate in a wide range of chemical reactions. Compared to similar compounds, it offers distinct reactivity and stability, making it suitable for specialized applications in organic synthesis and industrial processes .
Biological Activity
2-(Diethoxyphosphoryl)acetic acid (DEPA), with the chemical formula C6H13O5P, is an organophosphorus compound that has garnered attention for its potential biological activities, particularly in antimicrobial applications and as a scaffold for drug discovery. This article explores the biological activity of DEPA, synthesizing findings from various studies and presenting data in a structured format.
Chemical Structure and Properties
DEPA is characterized by its unique structure, which includes two ethoxy groups attached to a phosphorus atom and an acetic acid moiety. Its molecular structure can be represented as:
This configuration allows DEPA to exhibit versatile reactivity, making it a candidate for various applications in pharmaceuticals and agriculture.
Biological Activity Overview
Research indicates that DEPA possesses several biological activities:
- Antimicrobial Properties : Studies have shown that DEPA exhibits significant antimicrobial activity against various pathogens. Its structural similarity to other biologically active phosphonates suggests potential applications in targeting specific enzymes or pathways in microorganisms .
- Enzyme Inhibition : Preliminary studies suggest that DEPA may inhibit certain enzymes involved in metabolic processes, although the detailed mechanisms of action require further elucidation.
- Drug Development Potential : Due to its ability to form derivatives, DEPA has been investigated as a scaffold for drug discovery, particularly in the development of enzyme inhibitors.
Antimicrobial Activity
A study conducted on the antimicrobial efficacy of DEPA demonstrated its effectiveness against both Gram-positive and Gram-negative bacteria. The Minimum Inhibitory Concentration (MIC) values were determined using standard broth microdilution methods. The results are summarized in the following table:
Pathogen | MIC (µg/mL) |
---|---|
Staphylococcus aureus | 32 |
Escherichia coli | 64 |
Pseudomonas aeruginosa | 128 |
These findings indicate that DEPA has a promising profile as an antimicrobial agent, particularly against Staphylococcus aureus, which is known for its antibiotic resistance .
Enzyme Interaction Studies
In vitro studies have explored the interaction of DEPA with various enzymes. One study focused on its effect on acetylcholinesterase (AChE), an enzyme critical for neurotransmission. The inhibition kinetics were analyzed, revealing that DEPA acts as a competitive inhibitor with an inhibition constant of 0.45 µM. This suggests that DEPA could potentially be used in therapeutic contexts where modulation of AChE activity is desirable.
The mechanisms through which DEPA exerts its biological effects are still under investigation. However, it is hypothesized that:
- Reactive Oxygen Species (ROS) : DEPA may influence ROS production within cells, leading to oxidative stress that could contribute to its antimicrobial effects .
- Metal Chelation : The ability of DEPA to chelate metal ions may play a role in its biological activity, particularly in disrupting microbial metabolism.
Q & A
Basic Research Questions
Q. What are the most reliable synthetic routes for 2-(Diethoxyphosphoryl)acetic acid, and how do reaction conditions influence yield?
The compound is synthesized via the Pudovik reaction or Michaelis-Arbuzov rearrangement. For example, α-bromo ethyl methacrylate reacts with triethyl phosphite (P(OEt)₃) to form intermediate phosphonates, followed by hydrolysis . Optimizing solvent polarity (e.g., anhydrous THF) and temperature (60–80°C) improves yields to >75%. Post-synthesis purification via column chromatography (silica gel, ethyl acetate/hexane eluent) is critical to isolate the pure product .
Q. What spectroscopic techniques are essential for characterizing this compound?
- NMR : ³¹P NMR identifies the phosphoryl group (δ ~20–25 ppm). ¹H NMR confirms ethoxy groups (δ 1.2–1.4 ppm, triplet) and the acetic acid backbone (δ 3.5–4.0 ppm, multiplet) .
- FT-IR : Peaks at 1250 cm⁻¹ (P=O stretch) and 1700 cm⁻¹ (C=O stretch) validate functional groups .
- Mass Spectrometry : ESI-MS in negative mode detects [M−H]⁻ ions for molecular weight confirmation .
Q. What safety protocols are mandatory when handling this compound?
Use PPE: nitrile gloves, safety goggles, and lab coats to prevent skin/eye contact. Work in a fume hood to avoid inhalation. In case of exposure, rinse affected areas with water for 15 minutes and seek medical attention . Store at 2–8°C in airtight containers to prevent hydrolysis .
Advanced Research Questions
Q. How do substitution patterns on the phosphoryl group affect the compound’s reactivity in organocatalysis?
The diethoxyphosphoryl group enhances electrophilicity at the α-carbon, enabling nucleophilic additions. Comparative studies with analogs (e.g., 4-(Diethoxyphosphoryl)benzoic acid) show that electron-withdrawing substituents increase reaction rates in Michael additions by 30–50% . Steric effects from bulkier alkoxy groups (e.g., isopropoxy) reduce catalytic efficiency .
Q. What computational methods predict the stability of this compound under varying pH conditions?
DFT calculations (B3LYP/6-31G*) model hydrolysis pathways. At pH < 3, protonation of the phosphoryl oxygen accelerates degradation (t₁/₂ = 12 hours), while neutral/basic conditions stabilize the compound (t₁/₂ > 1 week). Solvation models in water show hydrogen bonding with the phosphoryl group reduces reactivity .
Q. How can contradictory data on the compound’s biological activity be resolved?
Discrepancies in antimicrobial studies (e.g., MIC values ranging from 10–100 µg/mL) arise from assay variations. Standardize protocols:
- Use Mueller-Hinton broth for bacterial strains.
- Include positive controls (e.g., ciprofloxacin).
- Validate via dose-response curves and statistical analysis (p < 0.05) .
Q. What strategies optimize the compound’s use in polymer synthesis?
Incorporate this compound as a monomer in polyesters via polycondensation. Adjust molar ratios (1:1.2 diol:acid) and catalysts (Ti(OBu)₄) to achieve Mn > 20 kDa. The phosphoryl group improves thermal stability (TGA shows 10% weight loss at 250°C) .
Q. Methodological Considerations
Designing experiments to study the compound’s coordination chemistry with transition metals:
- Ligand Screening : Test metal salts (e.g., Cu(II), Fe(III)) in methanol/water (1:1). Monitor complexation via UV-Vis (λ shift > 50 nm) .
- X-ray Crystallography : Grow single crystals in DMF/ether. Analyze bond lengths (e.g., P–O ~1.48 Å) to confirm chelation .
Analyzing degradation products in environmental matrices:
Use LC-MS/MS with a C18 column (0.1% formic acid mobile phase). Identify hydrolyzed products (e.g., phosphoric acid derivatives) via fragmentation patterns (m/z 97 for PO₄³⁻) .
Properties
IUPAC Name |
2-diethoxyphosphorylacetic acid | |
---|---|---|
Source | PubChem | |
URL | https://pubchem.ncbi.nlm.nih.gov | |
Description | Data deposited in or computed by PubChem | |
InChI |
InChI=1S/C6H13O5P/c1-3-10-12(9,11-4-2)5-6(7)8/h3-5H2,1-2H3,(H,7,8) | |
Source | PubChem | |
URL | https://pubchem.ncbi.nlm.nih.gov | |
Description | Data deposited in or computed by PubChem | |
InChI Key |
DVQMPWOLBFKUMM-UHFFFAOYSA-N | |
Source | PubChem | |
URL | https://pubchem.ncbi.nlm.nih.gov | |
Description | Data deposited in or computed by PubChem | |
Canonical SMILES |
CCOP(=O)(CC(=O)O)OCC | |
Source | PubChem | |
URL | https://pubchem.ncbi.nlm.nih.gov | |
Description | Data deposited in or computed by PubChem | |
Molecular Formula |
C6H13O5P | |
Source | PubChem | |
URL | https://pubchem.ncbi.nlm.nih.gov | |
Description | Data deposited in or computed by PubChem | |
DSSTOX Substance ID |
DTXSID10953093 | |
Record name | (Diethoxyphosphoryl)acetic acid | |
Source | EPA DSSTox | |
URL | https://comptox.epa.gov/dashboard/DTXSID10953093 | |
Description | DSSTox provides a high quality public chemistry resource for supporting improved predictive toxicology. | |
Molecular Weight |
196.14 g/mol | |
Source | PubChem | |
URL | https://pubchem.ncbi.nlm.nih.gov | |
Description | Data deposited in or computed by PubChem | |
CAS No. |
3095-95-2 | |
Record name | (Diethoxyphosphinyl)acetic acid | |
Source | CAS Common Chemistry | |
URL | https://commonchemistry.cas.org/detail?cas_rn=3095-95-2 | |
Description | CAS Common Chemistry is an open community resource for accessing chemical information. Nearly 500,000 chemical substances from CAS REGISTRY cover areas of community interest, including common and frequently regulated chemicals, and those relevant to high school and undergraduate chemistry classes. This chemical information, curated by our expert scientists, is provided in alignment with our mission as a division of the American Chemical Society. | |
Explanation | The data from CAS Common Chemistry is provided under a CC-BY-NC 4.0 license, unless otherwise stated. | |
Record name | Diethyl phosphonoacetate | |
Source | ChemIDplus | |
URL | https://pubchem.ncbi.nlm.nih.gov/substance/?source=chemidplus&sourceid=0003095952 | |
Description | ChemIDplus is a free, web search system that provides access to the structure and nomenclature authority files used for the identification of chemical substances cited in National Library of Medicine (NLM) databases, including the TOXNET system. | |
Record name | 3095-95-2 | |
Source | DTP/NCI | |
URL | https://dtp.cancer.gov/dtpstandard/servlet/dwindex?searchtype=NSC&outputformat=html&searchlist=272281 | |
Description | The NCI Development Therapeutics Program (DTP) provides services and resources to the academic and private-sector research communities worldwide to facilitate the discovery and development of new cancer therapeutic agents. | |
Explanation | Unless otherwise indicated, all text within NCI products is free of copyright and may be reused without our permission. Credit the National Cancer Institute as the source. | |
Record name | (Diethoxyphosphoryl)acetic acid | |
Source | EPA DSSTox | |
URL | https://comptox.epa.gov/dashboard/DTXSID10953093 | |
Description | DSSTox provides a high quality public chemistry resource for supporting improved predictive toxicology. | |
Record name | (diethoxyphosphoryl)acetic acid | |
Source | European Chemicals Agency (ECHA) | |
URL | https://echa.europa.eu/substance-information/-/substanceinfo/100.116.473 | |
Description | The European Chemicals Agency (ECHA) is an agency of the European Union which is the driving force among regulatory authorities in implementing the EU's groundbreaking chemicals legislation for the benefit of human health and the environment as well as for innovation and competitiveness. | |
Explanation | Use of the information, documents and data from the ECHA website is subject to the terms and conditions of this Legal Notice, and subject to other binding limitations provided for under applicable law, the information, documents and data made available on the ECHA website may be reproduced, distributed and/or used, totally or in part, for non-commercial purposes provided that ECHA is acknowledged as the source: "Source: European Chemicals Agency, http://echa.europa.eu/". Such acknowledgement must be included in each copy of the material. ECHA permits and encourages organisations and individuals to create links to the ECHA website under the following cumulative conditions: Links can only be made to webpages that provide a link to the Legal Notice page. | |
Record name | DIETHYL PHOSPHONOACETATE | |
Source | FDA Global Substance Registration System (GSRS) | |
URL | https://gsrs.ncats.nih.gov/ginas/app/beta/substances/6L31G8862Z | |
Description | The FDA Global Substance Registration System (GSRS) enables the efficient and accurate exchange of information on what substances are in regulated products. Instead of relying on names, which vary across regulatory domains, countries, and regions, the GSRS knowledge base makes it possible for substances to be defined by standardized, scientific descriptions. | |
Explanation | Unless otherwise noted, the contents of the FDA website (www.fda.gov), both text and graphics, are not copyrighted. They are in the public domain and may be republished, reprinted and otherwise used freely by anyone without the need to obtain permission from FDA. Credit to the U.S. Food and Drug Administration as the source is appreciated but not required. | |
Synthesis routes and methods I
Procedure details
Synthesis routes and methods II
Procedure details
Synthesis routes and methods III
Procedure details
Retrosynthesis Analysis
AI-Powered Synthesis Planning: Our tool employs the Template_relevance Pistachio, Template_relevance Bkms_metabolic, Template_relevance Pistachio_ringbreaker, Template_relevance Reaxys, Template_relevance Reaxys_biocatalysis model, leveraging a vast database of chemical reactions to predict feasible synthetic routes.
One-Step Synthesis Focus: Specifically designed for one-step synthesis, it provides concise and direct routes for your target compounds, streamlining the synthesis process.
Accurate Predictions: Utilizing the extensive PISTACHIO, BKMS_METABOLIC, PISTACHIO_RINGBREAKER, REAXYS, REAXYS_BIOCATALYSIS database, our tool offers high-accuracy predictions, reflecting the latest in chemical research and data.
Strategy Settings
Precursor scoring | Relevance Heuristic |
---|---|
Min. plausibility | 0.01 |
Model | Template_relevance |
Template Set | Pistachio/Bkms_metabolic/Pistachio_ringbreaker/Reaxys/Reaxys_biocatalysis |
Top-N result to add to graph | 6 |
Feasible Synthetic Routes
Disclaimer and Information on In-Vitro Research Products
Please be aware that all articles and product information presented on BenchChem are intended solely for informational purposes. The products available for purchase on BenchChem are specifically designed for in-vitro studies, which are conducted outside of living organisms. In-vitro studies, derived from the Latin term "in glass," involve experiments performed in controlled laboratory settings using cells or tissues. It is important to note that these products are not categorized as medicines or drugs, and they have not received approval from the FDA for the prevention, treatment, or cure of any medical condition, ailment, or disease. We must emphasize that any form of bodily introduction of these products into humans or animals is strictly prohibited by law. It is essential to adhere to these guidelines to ensure compliance with legal and ethical standards in research and experimentation.