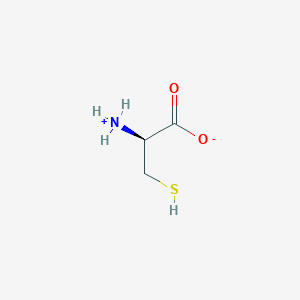
D-cysteine
Overview
Description
D-cysteine belongs to the class of organic compounds known as cysteine and derivatives . These compounds contain cysteine or a derivative thereof resulting from the reaction of cysteine at the amino group or the carboxy group, or from the replacement of any hydrogen of glycine by a heteroatom . Cysteine is chiral, and both D and L-cysteine are found in nature . Cysteine is a semiessential proteinogenic amino acid with the formula HOOC−CH (−NH2)−CH2−SH .
Synthesis Analysis
Cysteine biosynthesis is critically important for the production of an abundance of key metabolites in diverse pathways . It is synthesized in the human body when there is sufficient methionine available . The biosynthesis of cysteine is modified by regulators acting at the site of uptake and throughout the plant system .Chemical Reactions Analysis
Cysteine residues in proteins are subject to diverse redox chemistry . Oxidation of cysteine to S-nitrosocysteine, cysteine sulfenic and sulfinic acids, disulfides, and persulfides are a few prominent examples of these oxidative post-translational modifications . Cysteine often participates in enzymatic reactions as a nucleophile .Physical And Chemical Properties Analysis
This compound has a density of 1.3±0.1 g/cm^3, a boiling point of 293.9±35.0 °C at 760 mmHg, and a vapour pressure of 0.0±1.3 mmHg at 25°C . Its molar refractivity is 28.9±0.3 cm^3, and it has a molar volume of 90.8±3.0 cm^3 .Scientific Research Applications
Role in Human Health : D-Cysteine has been shown to play a significant role in human health. It is involved in the metabolic pathways of methionine, taurine, and glutathione (GSH), and may help combat chronic inflammation by enhancing antioxidant status (McPherson & Hardy, 2011).
Electrochemical Sensing Applications : this compound is a target for electrochemical sensing due to its importance in human health. Recent advancements in electrochemical strategies have enabled effective and reliable evaluation methods for detecting amino acids, including this compound, at trace and ultra-trace levels (Moulaee & Neri, 2021).
Neural Progenitor Cell Dynamics : this compound has been identified as an endogenous regulator of neural progenitor cell dynamics in the mammalian brain. It acts as a negative regulator of growth factor signaling during cortical development (Semenza et al., 2021).
Biomedical Imaging : this compound is used in bioluminescence imaging for detecting exogenous and endogenous cysteine in vitro and in vivo. This application aids in understanding the complex mechanisms of cysteine in various physiological and pathological processes (Hu et al., 2020).
Antibacterial Properties : Research has demonstrated that this compound exhibits distinct antibacterial activity against various pathogenic bacteria. It can disrupt the integrity of bacterial cell membranes, leading to cell death (Wang et al., 2019).
Mechanism of Action
Target of Action
D-Cysteine primarily targets the Carboxypeptidase A1 . It also interacts with D-amino acid oxidase (DAO) , which is expressed in Purkinje cells of primary cerebellar cultures . These targets play a crucial role in the metabolism and action of this compound.
Mode of Action
This compound interacts with its targets, leading to various biochemical reactions. For instance, this compound activates Chaperone-Mediated Autophagy (CMA) in Purkinje cells where DAO is expressed . This activation is believed to be mediated by the generation of hydrogen sulfide and Nrf2 activation .
Biochemical Pathways
This compound is involved in the cysteine metabolism pathway . It is synthesized from serine in a two-step pathway that begins with the O-acetylation of serine, followed by O-replacement of the acetyl group by sulfide or thiosulfate . This compound can also produce hydrogen sulfide in the cerebellum .
Pharmacokinetics
Studies on related compounds suggest that this compound is likely to be cleared primarily byhemodialysis . The biotransformation of this compound involves disulfide exchange with endogenous thiols, preserving the D-amino acid backbone .
Result of Action
This compound has been found to reduce the proliferation of cultured mouse embryonic neural progenitor cells (NPCs) by approximately 50% . This antiproliferative effect is mediated by the transcription factors FoxO1 and FoxO3a . Moreover, this compound is believed to enhance CMA activity via Nrf2 activation .
Action Environment
Environmental factors can influence the action of this compound. For instance, the status of glutathione (GSH) can positively impact the expression of Vitamin D metabolism genes, which could potentially influence the action of this compound . Additionally, annual seasonality has been found to be an important environmental factor influencing cytokine production, which could indirectly affect the action of this compound .
Safety and Hazards
Future Directions
The role of cysteine in tumor biology is being explored, which can help in the development of novel effective cancer therapies . There is also interest in the potential future directions of NAE-targeting drugs, which could include combination strategies with NAE inhibitors for cancer therapy, NAE agonists and their anticancer mechanisms, non-covalent NAE inhibitors, and applications for other diseases beyond cancer by targeting NAE .
properties
IUPAC Name |
(2S)-2-amino-3-sulfanylpropanoic acid | |
---|---|---|
Source | PubChem | |
URL | https://pubchem.ncbi.nlm.nih.gov | |
Description | Data deposited in or computed by PubChem | |
InChI |
InChI=1S/C3H7NO2S/c4-2(1-7)3(5)6/h2,7H,1,4H2,(H,5,6)/t2-/m1/s1 | |
Source | PubChem | |
URL | https://pubchem.ncbi.nlm.nih.gov | |
Description | Data deposited in or computed by PubChem | |
InChI Key |
XUJNEKJLAYXESH-UWTATZPHSA-N | |
Source | PubChem | |
URL | https://pubchem.ncbi.nlm.nih.gov | |
Description | Data deposited in or computed by PubChem | |
Canonical SMILES |
C(C(C(=O)O)N)S | |
Source | PubChem | |
URL | https://pubchem.ncbi.nlm.nih.gov | |
Description | Data deposited in or computed by PubChem | |
Isomeric SMILES |
C([C@H](C(=O)O)N)S | |
Source | PubChem | |
URL | https://pubchem.ncbi.nlm.nih.gov | |
Description | Data deposited in or computed by PubChem | |
Molecular Formula |
C3H7NO2S | |
Source | PubChem | |
URL | https://pubchem.ncbi.nlm.nih.gov | |
Description | Data deposited in or computed by PubChem | |
DSSTOX Substance ID |
DTXSID60893798 | |
Record name | D-Cysteine | |
Source | EPA DSSTox | |
URL | https://comptox.epa.gov/dashboard/DTXSID60893798 | |
Description | DSSTox provides a high quality public chemistry resource for supporting improved predictive toxicology. | |
Molecular Weight |
121.16 g/mol | |
Source | PubChem | |
URL | https://pubchem.ncbi.nlm.nih.gov | |
Description | Data deposited in or computed by PubChem | |
Physical Description |
Solid | |
Record name | D-Cysteine | |
Source | Human Metabolome Database (HMDB) | |
URL | http://www.hmdb.ca/metabolites/HMDB0003417 | |
Description | The Human Metabolome Database (HMDB) is a freely available electronic database containing detailed information about small molecule metabolites found in the human body. | |
Explanation | HMDB is offered to the public as a freely available resource. Use and re-distribution of the data, in whole or in part, for commercial purposes requires explicit permission of the authors and explicit acknowledgment of the source material (HMDB) and the original publication (see the HMDB citing page). We ask that users who download significant portions of the database cite the HMDB paper in any resulting publications. | |
CAS RN |
921-01-7 | |
Record name | D-Cysteine | |
Source | CAS Common Chemistry | |
URL | https://commonchemistry.cas.org/detail?cas_rn=921-01-7 | |
Description | CAS Common Chemistry is an open community resource for accessing chemical information. Nearly 500,000 chemical substances from CAS REGISTRY cover areas of community interest, including common and frequently regulated chemicals, and those relevant to high school and undergraduate chemistry classes. This chemical information, curated by our expert scientists, is provided in alignment with our mission as a division of the American Chemical Society. | |
Explanation | The data from CAS Common Chemistry is provided under a CC-BY-NC 4.0 license, unless otherwise stated. | |
Record name | Cysteine, D- | |
Source | ChemIDplus | |
URL | https://pubchem.ncbi.nlm.nih.gov/substance/?source=chemidplus&sourceid=0000921017 | |
Description | ChemIDplus is a free, web search system that provides access to the structure and nomenclature authority files used for the identification of chemical substances cited in National Library of Medicine (NLM) databases, including the TOXNET system. | |
Record name | D-Cysteine | |
Source | DrugBank | |
URL | https://www.drugbank.ca/drugs/DB03201 | |
Description | The DrugBank database is a unique bioinformatics and cheminformatics resource that combines detailed drug (i.e. chemical, pharmacological and pharmaceutical) data with comprehensive drug target (i.e. sequence, structure, and pathway) information. | |
Explanation | Creative Common's Attribution-NonCommercial 4.0 International License (http://creativecommons.org/licenses/by-nc/4.0/legalcode) | |
Record name | D-Cysteine | |
Source | EPA DSSTox | |
URL | https://comptox.epa.gov/dashboard/DTXSID60893798 | |
Description | DSSTox provides a high quality public chemistry resource for supporting improved predictive toxicology. | |
Record name | D-cysteine | |
Source | European Chemicals Agency (ECHA) | |
URL | https://echa.europa.eu/substance-information/-/substanceinfo/100.011.875 | |
Description | The European Chemicals Agency (ECHA) is an agency of the European Union which is the driving force among regulatory authorities in implementing the EU's groundbreaking chemicals legislation for the benefit of human health and the environment as well as for innovation and competitiveness. | |
Explanation | Use of the information, documents and data from the ECHA website is subject to the terms and conditions of this Legal Notice, and subject to other binding limitations provided for under applicable law, the information, documents and data made available on the ECHA website may be reproduced, distributed and/or used, totally or in part, for non-commercial purposes provided that ECHA is acknowledged as the source: "Source: European Chemicals Agency, http://echa.europa.eu/". Such acknowledgement must be included in each copy of the material. ECHA permits and encourages organisations and individuals to create links to the ECHA website under the following cumulative conditions: Links can only be made to webpages that provide a link to the Legal Notice page. | |
Record name | D-CYSTEINE | |
Source | FDA Global Substance Registration System (GSRS) | |
URL | https://gsrs.ncats.nih.gov/ginas/app/beta/substances/IU8WN3PPI2 | |
Description | The FDA Global Substance Registration System (GSRS) enables the efficient and accurate exchange of information on what substances are in regulated products. Instead of relying on names, which vary across regulatory domains, countries, and regions, the GSRS knowledge base makes it possible for substances to be defined by standardized, scientific descriptions. | |
Explanation | Unless otherwise noted, the contents of the FDA website (www.fda.gov), both text and graphics, are not copyrighted. They are in the public domain and may be republished, reprinted and otherwise used freely by anyone without the need to obtain permission from FDA. Credit to the U.S. Food and Drug Administration as the source is appreciated but not required. | |
Record name | D-Cysteine | |
Source | Human Metabolome Database (HMDB) | |
URL | http://www.hmdb.ca/metabolites/HMDB0003417 | |
Description | The Human Metabolome Database (HMDB) is a freely available electronic database containing detailed information about small molecule metabolites found in the human body. | |
Explanation | HMDB is offered to the public as a freely available resource. Use and re-distribution of the data, in whole or in part, for commercial purposes requires explicit permission of the authors and explicit acknowledgment of the source material (HMDB) and the original publication (see the HMDB citing page). We ask that users who download significant portions of the database cite the HMDB paper in any resulting publications. | |
Retrosynthesis Analysis
AI-Powered Synthesis Planning: Our tool employs the Template_relevance Pistachio, Template_relevance Bkms_metabolic, Template_relevance Pistachio_ringbreaker, Template_relevance Reaxys, Template_relevance Reaxys_biocatalysis model, leveraging a vast database of chemical reactions to predict feasible synthetic routes.
One-Step Synthesis Focus: Specifically designed for one-step synthesis, it provides concise and direct routes for your target compounds, streamlining the synthesis process.
Accurate Predictions: Utilizing the extensive PISTACHIO, BKMS_METABOLIC, PISTACHIO_RINGBREAKER, REAXYS, REAXYS_BIOCATALYSIS database, our tool offers high-accuracy predictions, reflecting the latest in chemical research and data.
Strategy Settings
Precursor scoring | Relevance Heuristic |
---|---|
Min. plausibility | 0.01 |
Model | Template_relevance |
Template Set | Pistachio/Bkms_metabolic/Pistachio_ringbreaker/Reaxys/Reaxys_biocatalysis |
Top-N result to add to graph | 6 |
Feasible Synthetic Routes
Q & A
Q1: What is the molecular formula and weight of D-cysteine?
A1: this compound shares the same molecular formula and weight as its L-enantiomer: C3H7NO2S, with a molecular weight of 121.16 g/mol.
Q2: Are there any specific spectroscopic data available for this compound?
A2: While specific spectroscopic data for this compound might be found in specialized databases, it's important to note that it shares the same chemical structure with L-cysteine, except for its chirality. Therefore, their spectroscopic properties (NMR, IR, etc.) would be largely identical. Techniques like chiral chromatography are necessary to differentiate between the two enantiomers.
Q3: How does this compound differ from L-cysteine in biological systems?
A3: Although structurally similar, this compound exhibits distinct biological activities compared to L-cysteine. For instance, this compound acts as a neuromodulator and shows potent neuroprotective activity in cerebellar primary neurons, unlike L-cysteine, which can be neurotoxic [].
Q4: What is the role of this compound in neural progenitor cell (NPC) dynamics?
A4: this compound functions as a negative regulator of growth factor signaling during cortical development. It has been shown to reduce the proliferation of cultured mouse embryonic NPCs by approximately 50% []. This effect is mediated by the transcription factors FoxO1 and FoxO3a [].
Q5: How does this compound affect chaperone-mediated autophagy (CMA) in cerebellar Purkinje cells?
A5: this compound, when metabolized by D-amino acid oxidase (DAO), generates hydrogen sulfide (H2S). This H2S production in the cerebellum leads to the activation of CMA in Purkinje cells []. This activation is mediated through Nuclear factor erythroid 2-related factor 2 (Nrf2) [].
Q6: Can this compound impact insulin production?
A6: Research suggests that this compound is present in the pancreas and plays a role in regulating insulin promoter methylation []. In studies with mice lacking serine racemase (SR), the enzyme responsible for this compound biosynthesis, pancreatic and plasma insulin levels were significantly elevated []. This suggests a regulatory role of this compound in insulin production.
Q7: How does this compound interact with myristoylated alanine-rich C-kinase substrate (MARCKS)?
A7: Studies indicate a potential interaction between this compound and MARCKS. This compound may bind to MARCKS, altering its phosphorylation state at specific serine residues (Ser 159/163) and influencing its translocation from the membrane [, ]. This interaction might be crucial for this compound's effects on NPC proliferation.
Q8: What is the role of this compound in hydrogen sulfide (H2S) production?
A8: this compound serves as a substrate for H2S production through a pathway involving DAO and 3MST [, ]. DAO converts this compound to 3-mercaptopyruvate, which is then utilized by 3MST to generate H2S. This pathway is particularly active in the cerebellum and kidneys, contributing to H2S-mediated physiological effects in these tissues.
Q9: How does this compound contribute to plant responses to environmental stress?
A9: Research suggests that this compound might play a role in plant stress responses by influencing reactive sulfur species (RSS) signaling []. In the water fern Azolla pinnata, this compound induced rapid root abscission, a response triggered by various environmental stresses []. This suggests a potential link between this compound, RSS, and plant adaptation to stress.
Q10: What is the therapeutic potential of this compound in treating opioid-induced respiratory depression?
A10: this compound ethyl ester (D-CYSee), a cell-permeant derivative of this compound, has shown promise in reversing the respiratory depressive effects of opioids like morphine in rats []. D-CYSee effectively reversed morphine-induced decreases in tidal volume, minute ventilation, and peak inspiratory flow without significantly impacting morphine's analgesic or sedative effects [].
Q11: Can this compound be used to prevent physical dependence on opioids?
A11: Studies in rats demonstrate that co-injection of D-CYSee or this compound ethyl amide with fentanyl significantly reduced withdrawal symptoms precipitated by naloxone, indicating a potential for preventing the development of physical dependence [].
Q12: How does this compound compare to L-cysteine in protecting against acetaminophen-induced liver injury?
A12: Unlike N-acetyl-L-cysteine, which effectively protects against acetaminophen-induced hepatotoxicity by increasing glutathione levels, N-acetyl-D-cysteine did not provide the same level of protection []. This suggests that the protective mechanism of N-acetyl-L-cysteine likely involves glutathione biosynthesis, which is not stimulated by the D-isomer.
Q13: Could this compound be used as a treatment for spinocerebellar ataxia (SCA)?
A13: Preclinical research shows that this compound administration improved motor function in a mouse model of SCA [, ]. This therapeutic benefit might be linked to the enhanced production of H2S from this compound in the cerebellum.
Q14: Does this compound have any potential in treating chronic kidney disease (CKD)?
A14: In a rat model of CKD, dietary supplementation with both L- and this compound during pregnancy prevented hypertension in the male offspring []. The protective effect was associated with reduced oxidative stress, rebalanced renin-angiotensin system (RAS), and altered gut microbiome composition [].
Q15: Can this compound be utilized in nanosensor applications?
A15: Functionalized CdS quantum dots (CdS-QDs) using this compound have been explored as nanosensors for detecting chiral molecules like D-methamphetamine []. The fluorescence of this compound functionalized CdS-QDs is selectively quenched by D-methamphetamine, enabling its detection and quantification.
Q16: What are the known toxicological effects of this compound?
A16: In a 4-week repeated dose toxicity study in rats, this compound administered orally at 2,000 mg/kg/day caused anemia, renal injuries, and sperm granuloma in the epididymis []. One rat in this group died due to renal failure, suggesting potential toxicity at high doses []. The no-observed-adverse-effect level (NOAEL) for this compound was determined to be 500 mg/kg/day under the study conditions [].
Disclaimer and Information on In-Vitro Research Products
Please be aware that all articles and product information presented on BenchChem are intended solely for informational purposes. The products available for purchase on BenchChem are specifically designed for in-vitro studies, which are conducted outside of living organisms. In-vitro studies, derived from the Latin term "in glass," involve experiments performed in controlled laboratory settings using cells or tissues. It is important to note that these products are not categorized as medicines or drugs, and they have not received approval from the FDA for the prevention, treatment, or cure of any medical condition, ailment, or disease. We must emphasize that any form of bodily introduction of these products into humans or animals is strictly prohibited by law. It is essential to adhere to these guidelines to ensure compliance with legal and ethical standards in research and experimentation.