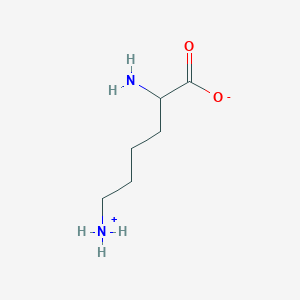
DL-Lysine
Overview
Description
DL-Lysine is a racemic mixture of the enantiomers D-lysine and L-lysine. It is synthetically produced and widely used in biochemical and pharmaceutical research. Key properties include:
- Chemical Formula: C₆H₁₄N₂O₂·HCl (hydrochloride salt form) .
- Molecular Weight: 182.65 g/mol .
- Purity: ≥98% in commercial preparations, with applications ranging from protein synthesis studies to drug formulation .
- Pharmaceutical Use: this compound is a component of Lysine Acetylsalicylate (LAS), a soluble aspirin derivative for intravenous pain management .
Preparation Methods
Chemical Synthesis of DL-Lysine
Racemization of L-Lysine Hydrochloride
The most common industrial method for this compound production involves racemizing L-lysine hydrochloride. As detailed in a patented process, L-lysine hydrochloride is dissolved in glacial acetic acid and reacted with salicylic aldehyde as a catalyst at elevated temperatures (95–105°C) under reflux for 6–8 hours . This reaction induces racemization, converting L-lysine into its D-enantiomer and yielding this compound hydrochloride with a purity exceeding 95% . Key parameters include:
-
Catalyst ratio : 0.1–0.15 parts salicylic aldehyde per part of L-lysine hydrochloride .
-
Solvent system : Glacial acetic acid in a 5:1 mass ratio to L-lysine hydrochloride .
-
Post-reaction processing : Centrifugation and drying to isolate this compound hydrochloride .
This method achieves yields of 95–97%, with the racemic product requiring subsequent resolution for enantiomer separation .
Resolution of this compound Hydrochloride
Diastereomeric Salt Formation with Chiral Resolving Agents
The resolution of this compound hydrochloride into its enantiomers typically employs chiral resolving agents. A patented technique uses L-(-)-camphorsulfonic acid to selectively crystallize the D-lysine enantiomer . The process involves:
-
Dissolution and heating : this compound hydrochloride is dissolved in water (6–7:1 mass ratio to resolving agent) and heated to 80–90°C .
-
Crystallization : Cooling to 5–10°C induces precipitation of the L-(-)-camphorsulfonic acid-D-lysine salt .
-
Washing : The solid is washed with 70–80% ethanol to remove residual L-lysine .
Table 1: Resolution Efficiency Under Varied Conditions
Enzymatic Optical Resolution
Recent advances explore enzymatic methods for resolving this compound. δ-Selenolysine derivatives, synthesized from DL-δ-hydroxylysine, undergo enzymatic hydrolysis using L- or D-aminoacylase to yield enantiomerically pure L- or D-lysine . For example:
-
Substrate : DL-δ-hydroxylysine acetylated at the ε-amino group .
-
Enzymes : L-aminoacylase preferentially hydrolyzes the L-enantiomer, leaving D-δ-hydroxylysine acetylated for subsequent isolation .
This method avoids harsh chemical conditions but requires costly enzymes and specialized handling of selenolysine intermediates .
Industrial-Scale Process Optimization
Desalination and Purification
Post-resolution, the D-lysine·L-CSA complex undergoes desalination via ion-exchange resins (e.g., Hydrogen 732) to remove camphorsulfonic acid . The eluate is concentrated and adjusted to pH 3–4 with hydrochloric acid, followed by decoloration using acid-activated carbon (3–5% w/v) at 45–55°C .
Table 2: Purification Metrics
Step | Conditions | Outcome |
---|---|---|
Desalination | Hydrogen 732 resin, 2N NH₃ | 90–95% acid removal |
Decoloration | 45–55°C, 0.5–1 h | 99% pigment removal |
Crystallization | HCl-adjusted pH 3–4 | 85–90% recovery |
Challenges and Innovations
Limitations of Current Methods
-
Resolution efficiency : Chemical methods yield ≤50% per cycle, necessitating multi-step recycling .
-
Cost of resolving agents : L-CSA accounts for 30–40% of production costs .
-
Environmental impact : Large volumes of ethanol and acetic acid require recovery systems .
Emerging Techniques
Chemical Reactions Analysis
Oxidation Reactions
DL-Lysine undergoes oxidation primarily at its amino groups:
- ε-Amino Group Oxidation : The ε-amino group is oxidized to form Nε-oxo derivatives (e.g., α-aminoadipate semialdehyde) using oxidizing agents like potassium permanganate (KMnO₄) or hydrogen peroxide (H₂O₂) under acidic conditions .
- α-Amino Group Oxidation : The α-amino group can be oxidized to form imines or nitriles under strong oxidative conditions .
Key Data:
Reagent/Conditions | Product | Reference |
---|---|---|
KMnO₄ (acidic) | Nε-oxo derivatives | |
H₂O₂ (catalytic Fe²⁺) | α-Aminoadipate semialdehyde |
Reduction Reactions
The ε-amino group of this compound can be reduced to form secondary amines or cyclic derivatives:
- Catalytic Hydrogenation : Using palladium or platinum catalysts, lysine is reduced to cadaverine (1,5-diaminopentane) .
- Sodium Borohydride (NaBH₄) : Reduces Schiff base intermediates to stable adducts .
Key Data:
Reagent/Conditions | Product | Reference |
---|---|---|
H₂/Pd catalyst | Cadaverine | |
NaBH₄ | Stable amine adducts |
Substitution Reactions
This compound’s amino groups participate in nucleophilic substitution:
- Acylation : Reacts with acyl chlorides (e.g., acetyl chloride) to form Nε-acetyllysine .
- Alkylation : Forms Nε-methyllysine with methyl iodide .
Key Data:
Reagent/Conditions | Product | Reference |
---|---|---|
Acetyl chloride | Nε-acetyllysine | |
Methyl iodide | Nε-methyllysine |
Decarboxylation Reactions
The carboxyl group of this compound undergoes enzymatic or chemical decarboxylation:
- Enzymatic Decarboxylation : Lysine decarboxylase (with pyridoxal phosphate, PLP) converts lysine to cadaverine , releasing CO₂ .
- Thermal Decarboxylation : At high temperatures (>200°C), this compound forms cyclic amines .
Key Data:
Conditions | Product | Reference |
---|---|---|
PLP, 37°C | Cadaverine + CO₂ | |
220°C, inert atmosphere | Cyclic amines |
Schiff Base Formation
The ε-amino group reacts with aldehydes or ketones to form Schiff bases:
- Formaldehyde Reaction : Produces Nε-methylenelysine , a reversible intermediate .
- Biocatalytic Applications : Schiff bases act as intermediates in enzyme-catalyzed processes (e.g., aldehyde synthesis) .
Key Data:
Aldehyde/Ketone | Product | Reference |
---|---|---|
Formaldehyde | Nε-methylenelysine | |
Glycolaldehyde | Stable Schiff base |
Conjugation Reactions
This compound forms conjugates with carbonyl-containing metabolites:
- Amadori Reaction : Reacts with glucose to form fructoselysine .
- Acetylation : Conjugates with acetyl-CoA to form Nε-acetyllysine , a key histone modification .
Key Data:
Substrate | Product | Reference |
---|---|---|
Glucose | Fructoselysine | |
Acetyl-CoA | Nε-acetyllysine |
Thermochemical Data
Reaction enthalpies for this compound-derived processes:
Reaction | ΔH (kJ/mol) | Method | Reference |
---|---|---|---|
Na⁺ + this compound → (Na⁺ - this compound) | >213 | CIDC |
Scientific Research Applications
DL-Lysine has a wide range of applications in scientific research:
Chemistry: Used as a building block for the synthesis of various chemical compounds.
Biology: Employed in studies related to protein structure and function.
Medicine: Investigated for its potential therapeutic effects, including antiviral and anti-inflammatory properties.
Mechanism of Action
DL-Lysine exerts its effects through various molecular pathways. In biological systems, it can be incorporated into proteins, affecting their structure and function. Additionally, this compound can inhibit the replication of certain viruses by interfering with their ability to utilize arginine, an amino acid essential for viral replication . The compound also participates in the regulation of gene expression through histone modifications, impacting the epigenome.
Comparison with Similar Compounds
Structural and Physical Properties
DL-Lysine differs structurally from its enantiomers and other amino acids due to its racemic nature. Comparative data are summarized below:
Metabolic and Pharmacokinetic Differences
Uptake and Clearance :
- In dogs, the D-isomer in this compound contributes to prolonged C14 excretion (~10% extra elimination between 2–10 hours) compared to pure L-Lysine .
Functional and Biochemical Roles
- Enzyme Inhibition: this compound and L-arginine are more effective than D-alanine or glycine in protecting against endogenous phosphatase inactivation .
- Synthesis and Resolution : Immobilized trypsin resolves this compound methyl ester into L-lysine and D-lysine methyl ester, highlighting enzymatic stereospecificity .
Key Research Findings
- Metabolic Inertness : this compound’s racemic nature reduces its metabolic utility in certain contexts (e.g., rumen fermentation) compared to single enantiomers .
- Structural Flexibility : Racemic this compound adapts to centrosymmetric aggregation in crystals, enabling unique material applications .
- Clinical Utility : LAS leverages this compound’s solubility for rapid drug delivery, a property absent in pure L- or D-forms .
Biological Activity
DL-Lysine, a racemic mixture of the amino acids L-lysine and D-lysine, has garnered attention for its diverse biological activities. This article explores the biological activity of this compound, focusing on its antimicrobial properties, immunomodulatory effects, and potential applications in cancer therapy.
Overview of this compound
This compound is an essential amino acid that plays a crucial role in protein synthesis and various metabolic processes. While L-lysine is biologically active in humans, D-lysine is typically less active but can exhibit unique properties when incorporated into peptides or proteins.
Antimicrobial Activity
Research indicates that the incorporation of D-lysine into antimicrobial peptides (AMPs) can enhance their efficacy while reducing toxicity to eukaryotic cells. For instance, a study on modified cecropin A and melittin hybrid peptides demonstrated that introducing D-lysine residues resulted in:
- Reduced Toxicity : D-lysine substitutions decreased the cytotoxic effects on human erythrocytes and murine macrophages.
- Maintained Antimicrobial Activity : Although there was a moderate reduction in antimicrobial activity against Staphylococcus aureus, the overall selectivity towards bacterial cells improved significantly .
Table 1: Effects of D-Lysine Substitutions on Antimicrobial Activity
Peptide Variant | Antimicrobial Activity | Toxicity to Eukaryotic Cells | Selectivity Index |
---|---|---|---|
CM15 | High | Moderate | 1.5 |
D1,13 | Moderate | Low | 3.0 |
D3,14 | Moderate | Very Low | 5.0 |
Data derived from studies evaluating the impact of D-lysine on peptide structure and function .
Immunomodulatory Effects
This compound has also been studied for its immunomodulatory properties. In animal models, it has been shown to influence immune responses positively:
- Enhanced Immune Response : In studies involving rabies vaccination, this compound treatment improved the humoral immune response by increasing antibody production against the rabies virus .
- Cytokine Production : Treatments with this compound have been associated with increased levels of gamma-interferon (IFN-γ), indicating a potential role in enhancing cellular immunity .
Anti-Cancer Properties
Recent studies have explored the anti-cancer potential of D-lysine-modified peptides. For example:
- Phylloseptin-like Peptides : Modifications with D-lysines led to peptides exhibiting significant anti-proliferative activity against human lung cancer cell lines (H838 and H460). These modifications not only enhanced antimicrobial properties but also reduced cytotoxicity, suggesting a higher therapeutic index for potential drug development .
Case Study: Anti-Cancer Activity of D-Lysine Substituted Peptides
A study investigated the effects of a phylloseptin-like peptide modified with D-lysines:
- Findings :
- The modified peptide showed enhanced activity against multidrug-resistant strains of Staphylococcus aureus.
- It exhibited reduced hemolytic activity, indicating lower toxicity to human cells while maintaining antibacterial efficacy.
Q & A
Basic Research Questions
Q. How can researchers determine the enantiomeric purity of DL-Lysine in synthesized samples?
Methodological Answer: Enantiomeric purity can be assessed using chiral high-performance liquid chromatography (HPLC) with a chiral stationary phase (e.g., cyclodextrin-based columns). The separation exploits differential interactions between D- and L-lysine enantiomers and the chiral selector. Alternatively, enzymatic assays using L-lysine-specific decarboxylase can selectively quantify L-lysine, with residual D-lysine measured via spectrophotometry .
Q. What analytical techniques are recommended for quantifying this compound in complex biological matrices?
Methodological Answer: A validated spectrophotometric method involves reacting this compound with sodium tetraborate and sodium nitroprussiate under alkaline conditions, forming a colored complex with maximum absorbance at 545 nm. This method is specific and avoids interference from common additives like vitamins or amino acids . For higher sensitivity, LC-MS/MS with isotope-labeled internal standards (e.g., ¹³C-lysine) provides precise quantification in tissues or plasma .
Q. How should experimental protocols be designed to synthesize this compound derivatives with minimal racemization?
Methodological Answer: To minimize racemization during synthesis:
- Use mild coupling reagents (e.g., HOBt/DIC) in peptide synthesis.
- Maintain pH below 8.0 during reactions to avoid base-induced epimerization.
- Monitor reaction progress with circular dichroism (CD) spectroscopy to detect optical activity changes .
Advanced Research Questions
Q. What enzymatic strategies enable the selective production of D-Lysine from this compound mixtures?
Methodological Answer: A two-step enzymatic cascade can achieve this:
- Racemization: Use whole-cell systems expressing amino acid racemase (AAR) to convert L-lysine to this compound.
- Decarboxylation: Add lysine decarboxylase (LDC) to selectively convert L-lysine to 1,5-pentanediamine, leaving D-lysine intact. D-Lysine is then isolated via isoelectric crystallization (pI = 9.7) and ion-exchange chromatography .
Q. How can researchers resolve discrepancies in COX inhibition data for this compound derivatives?
Methodological Answer: Discrepancies often arise from assay conditions:
- Enzyme Source: Use recombinant human COX-1/2 isoforms instead of non-human extracts to avoid interspecies variability.
- Inhibition Metrics: Report both IC₅₀ (half-maximal inhibition) and kinetic parameters (e.g., Ki) to distinguish competitive vs. non-competitive mechanisms.
- Control Experiments: Include aspirin (a known COX inhibitor) and validate results across multiple assays (e.g., fluorometric vs. ELISA-based prostaglandin quantification) .
Q. What metabolic pathways interact with this compound in mammalian systems, and how can these be modeled in vitro?
Methodological Answer: this compound is metabolized via the saccharopine pathway to acetyl-CoA. To model this:
- Use ¹³C-labeled this compound in tracer studies with GC-MS to track carbon flux in cultured hepatocytes.
- Knock down key enzymes (e.g., α-aminoadipic semialdehyde synthase) using siRNA to identify rate-limiting steps .
Q. How do structural modifications of this compound (e.g., acetylation) alter its physicochemical properties?
Methodological Answer: Acetylation of the ε-amino group (e.g., Aspirin this compound) increases hydrophilicity and stability. Characterize modifications using:
- NMR Spectroscopy: ¹H and ¹³C NMR to confirm acetylation sites.
- Thermogravimetric Analysis (TGA): Assess thermal stability (e.g., decomposition at ~267°C for this compound·HCl) .
Q. Data Contradiction Analysis
Q. Why do studies report conflicting bioactivity results for this compound in cancer models?
Methodological Answer: Variations arise from:
- Cell Line Specificity: this compound derivatives may target COX-2 in HT-29 colon cancer cells but not in MCF-7 breast cancer cells.
- Dosage Regimens: Anti-proliferative effects are often dose-dependent; subtherapeutic concentrations may paradoxically enhance growth.
- Metabolic Context: Hypoxic conditions in tumors alter lysine uptake and utilization, confounding in vivo vs. in vitro results .
Properties
IUPAC Name |
2,6-diaminohexanoic acid | |
---|---|---|
Source | PubChem | |
URL | https://pubchem.ncbi.nlm.nih.gov | |
Description | Data deposited in or computed by PubChem | |
InChI |
InChI=1S/C6H14N2O2/c7-4-2-1-3-5(8)6(9)10/h5H,1-4,7-8H2,(H,9,10) | |
Source | PubChem | |
URL | https://pubchem.ncbi.nlm.nih.gov | |
Description | Data deposited in or computed by PubChem | |
InChI Key |
KDXKERNSBIXSRK-UHFFFAOYSA-N | |
Source | PubChem | |
URL | https://pubchem.ncbi.nlm.nih.gov | |
Description | Data deposited in or computed by PubChem | |
Canonical SMILES |
C(CCN)CC(C(=O)O)N | |
Source | PubChem | |
URL | https://pubchem.ncbi.nlm.nih.gov | |
Description | Data deposited in or computed by PubChem | |
Molecular Formula |
C6H14N2O2 | |
Source | PubChem | |
URL | https://pubchem.ncbi.nlm.nih.gov | |
Description | Data deposited in or computed by PubChem | |
Related CAS |
26714-32-9 | |
Record name | Lysine, homopolymer | |
Source | CAS Common Chemistry | |
URL | https://commonchemistry.cas.org/detail?cas_rn=26714-32-9 | |
Description | CAS Common Chemistry is an open community resource for accessing chemical information. Nearly 500,000 chemical substances from CAS REGISTRY cover areas of community interest, including common and frequently regulated chemicals, and those relevant to high school and undergraduate chemistry classes. This chemical information, curated by our expert scientists, is provided in alignment with our mission as a division of the American Chemical Society. | |
Explanation | The data from CAS Common Chemistry is provided under a CC-BY-NC 4.0 license, unless otherwise stated. | |
DSSTOX Substance ID |
DTXSID90859004 | |
Record name | DL-Lysine | |
Source | EPA DSSTox | |
URL | https://comptox.epa.gov/dashboard/DTXSID90859004 | |
Description | DSSTox provides a high quality public chemistry resource for supporting improved predictive toxicology. | |
Molecular Weight |
146.19 g/mol | |
Source | PubChem | |
URL | https://pubchem.ncbi.nlm.nih.gov | |
Description | Data deposited in or computed by PubChem | |
CAS No. |
70-54-2 | |
Record name | (±)-Lysine | |
Source | CAS Common Chemistry | |
URL | https://commonchemistry.cas.org/detail?cas_rn=70-54-2 | |
Description | CAS Common Chemistry is an open community resource for accessing chemical information. Nearly 500,000 chemical substances from CAS REGISTRY cover areas of community interest, including common and frequently regulated chemicals, and those relevant to high school and undergraduate chemistry classes. This chemical information, curated by our expert scientists, is provided in alignment with our mission as a division of the American Chemical Society. | |
Explanation | The data from CAS Common Chemistry is provided under a CC-BY-NC 4.0 license, unless otherwise stated. | |
Record name | Lysine, DL- | |
Source | ChemIDplus | |
URL | https://pubchem.ncbi.nlm.nih.gov/substance/?source=chemidplus&sourceid=0000070542 | |
Description | ChemIDplus is a free, web search system that provides access to the structure and nomenclature authority files used for the identification of chemical substances cited in National Library of Medicine (NLM) databases, including the TOXNET system. | |
Record name | DL-Lysine | |
Source | EPA DSSTox | |
URL | https://comptox.epa.gov/dashboard/DTXSID90859004 | |
Description | DSSTox provides a high quality public chemistry resource for supporting improved predictive toxicology. | |
Record name | DL-lysine | |
Source | European Chemicals Agency (ECHA) | |
URL | https://echa.europa.eu/substance-information/-/substanceinfo/100.000.673 | |
Description | The European Chemicals Agency (ECHA) is an agency of the European Union which is the driving force among regulatory authorities in implementing the EU's groundbreaking chemicals legislation for the benefit of human health and the environment as well as for innovation and competitiveness. | |
Explanation | Use of the information, documents and data from the ECHA website is subject to the terms and conditions of this Legal Notice, and subject to other binding limitations provided for under applicable law, the information, documents and data made available on the ECHA website may be reproduced, distributed and/or used, totally or in part, for non-commercial purposes provided that ECHA is acknowledged as the source: "Source: European Chemicals Agency, http://echa.europa.eu/". Such acknowledgement must be included in each copy of the material. ECHA permits and encourages organisations and individuals to create links to the ECHA website under the following cumulative conditions: Links can only be made to webpages that provide a link to the Legal Notice page. | |
Record name | LYSINE, DL- | |
Source | FDA Global Substance Registration System (GSRS) | |
URL | https://gsrs.ncats.nih.gov/ginas/app/beta/substances/AI4RT59273 | |
Description | The FDA Global Substance Registration System (GSRS) enables the efficient and accurate exchange of information on what substances are in regulated products. Instead of relying on names, which vary across regulatory domains, countries, and regions, the GSRS knowledge base makes it possible for substances to be defined by standardized, scientific descriptions. | |
Explanation | Unless otherwise noted, the contents of the FDA website (www.fda.gov), both text and graphics, are not copyrighted. They are in the public domain and may be republished, reprinted and otherwise used freely by anyone without the need to obtain permission from FDA. Credit to the U.S. Food and Drug Administration as the source is appreciated but not required. | |
Retrosynthesis Analysis
AI-Powered Synthesis Planning: Our tool employs the Template_relevance Pistachio, Template_relevance Bkms_metabolic, Template_relevance Pistachio_ringbreaker, Template_relevance Reaxys, Template_relevance Reaxys_biocatalysis model, leveraging a vast database of chemical reactions to predict feasible synthetic routes.
One-Step Synthesis Focus: Specifically designed for one-step synthesis, it provides concise and direct routes for your target compounds, streamlining the synthesis process.
Accurate Predictions: Utilizing the extensive PISTACHIO, BKMS_METABOLIC, PISTACHIO_RINGBREAKER, REAXYS, REAXYS_BIOCATALYSIS database, our tool offers high-accuracy predictions, reflecting the latest in chemical research and data.
Strategy Settings
Precursor scoring | Relevance Heuristic |
---|---|
Min. plausibility | 0.01 |
Model | Template_relevance |
Template Set | Pistachio/Bkms_metabolic/Pistachio_ringbreaker/Reaxys/Reaxys_biocatalysis |
Top-N result to add to graph | 6 |
Feasible Synthetic Routes
Disclaimer and Information on In-Vitro Research Products
Please be aware that all articles and product information presented on BenchChem are intended solely for informational purposes. The products available for purchase on BenchChem are specifically designed for in-vitro studies, which are conducted outside of living organisms. In-vitro studies, derived from the Latin term "in glass," involve experiments performed in controlled laboratory settings using cells or tissues. It is important to note that these products are not categorized as medicines or drugs, and they have not received approval from the FDA for the prevention, treatment, or cure of any medical condition, ailment, or disease. We must emphasize that any form of bodily introduction of these products into humans or animals is strictly prohibited by law. It is essential to adhere to these guidelines to ensure compliance with legal and ethical standards in research and experimentation.